Flexibility benefits for Power System Resilience
Authors
E. HILLBERG - RISE, Sweden
I. OLEINIKOVA - NTNU, Norway
A. ILICETO - ETIP SNET, Italy

Summary
As cheap and affordable variable Renewable Energy Sources (vRES), such as wind farms and photovoltaics, are foreseen to dominate the future energy mix, the abundance of green electricity will allow the replacement of fossil fuels in sectors such as heating, cooling, industrial processes, and transport.
The intermittency and/or low controllability of vRES implies the significant systemic needs of flexible solutions; therefore, the developments of the energy system in general, and the power sector in particular, have led to significant innovation activities in the fields of power system flexibility. At the same time, the complexity and interdependencies of system components and multitude of actors increase the risks of service failures, thus raising needs of stronger and more flexible resilience means and countermeasures.
Power system resilience reflects the impact of severe and/or unpredictable events and is an overarching concept, covering the whole spectrum of the power system from design and investment decisions to planning, operations, maintenance, and asset management functions.
Flexibility concerns the power systems ability to manage changes; the future wide portfolio of flexibility means and their features can also improve the resilience characteristics of the broader view system of systems, provided that they are integrated in grid planning, grid operation, defence plans, and properly evaluated in the energy market design.
In this paper we are discussing on “How can flexibility also support resilience?”, considering the increased societal needs of a secure electricity supply.
Keywords
Flexibility - Resilience - Renewable Energy Sources - Extraordinary Events - Power System Operation - Power System Planning1. Introduction
If planning of the power systems does not provide adequate levels and availability of resources, especially in front of contingencies (i.e., unforeseen, rapid changes of operational conditions), existing resources may become insufficient to guarantee a stable operation and ensure the continuity of supply. The reinforcements and optimal utilisation of system resources are necessary to maintain the full functionalities of the increasingly complex and intertwined energy system (“system of systems”). Thus, the investment planning process is a first key step to ensure the adequacy of future electricity system, i.e., sufficient ability to accommodate the growth of variable generation from renewable energy sources and to cope with foreseeable contingencies.
For this aim, different sources of flexibility must be utilised, provided by the various equipment connected to the system, both power system and other coupled ones (gas, heat, transport). This requires an approach involving activities from the consumers and from actors in other energy systems, which can be utilised also to increase the resilience of power grids against a variety of future threats.
Generally, the electrical infrastructure is designed and operated aiming at the capability (until a certain level) of a quick and self-balancing reaction to possible threats of various nature. The progressive electrification, as one of the directions adopted for decarbonisation of other sectors, carries with it even greater challenges for maintaining the stability and continuity of electricity supply. On the other hand, the activation of consumers as well as the pursuit of energy efficiency and optimization show that in the future market, the use of sources of flexibility will play a significant role in the management of the power system.
Furthermore, a high level of flexibility and system integration will not be possible without deep digitalization of all energy sectors and processes. Consequently, the operational processes are requiring proper management in the handling of big data. Artificial intelligence, machine learning techniques and algorithms will be able to provide significant value and support to the energy sector. At the same time, the amount and the level of cyber threats increase due to the very high number of access points and interdependencies. The increased complexities thus require a shift of the centre of gravity of trust:
- from trust in the reliability and appropriateness of established human procedures
- to trust in procedures created, activated, and executed by automatised systems.
Therefore, both flexibility and resilience have to be addressed: flexibility for grid operation with high vRES penetration, starts being considered at the investment planning stage and resilience, based on reliability, risk analysis, system interactions analysis, within the perimeter of the electric power system.
2. Flexibility, resilience and extraordinary events
For the sake of clarity, commonly accepted definitions for flexibility and resilience are needed. In this section, simplicity is proposed in both these regards.
2.1. Defining Flexibility in the power system context
Flexibility of the power system concerns the power systems ability to manage changes [1], which can impact the preservation of a secure and reliable operation of the power system. Flexibility solutions provide support to the grid during normal operation, where the benefits provided by increased flexibility include decreasing of overall costs (including capex for asset base needs) and the overcoming of challenges related to for example grid congestion. Flexibility needs span the timescales from sub-seconds to seasons and years, on local and over-all system levels, as illustrated in Figure 1.
Solutions providing flexibility are especially relevant in cases with higher share of variable renewable energy sources. Flexibility solutions enable connected entities to support the grid as needed, utilising the abilities of available grid elements.
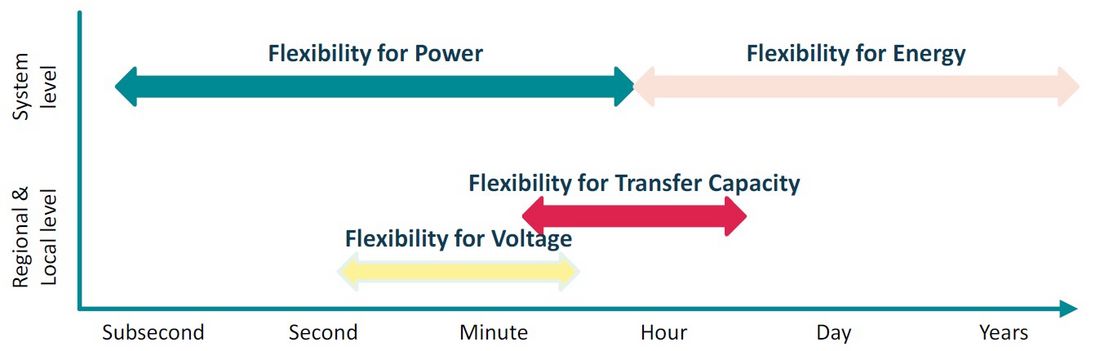
Figure 1 - Flexibility needs from spatial and temporal perspectives, from [1]
There are several definitions intending to describe the term flexibility, several of which listed in [1]. In CIGRE, two descriptions of flexibility may be notable:
- In [2], flexibility is described from the system planner’s perspective, as the ability to adapt the planned development of the power system to any change.
- While [3] describes the short-term flexibility focusing on its balancing function, as the capability to consume or produce electrical energy in response to changing power system conditions.
Most existing definitions have specific characteristics coming from different perspectives, as highlighted in [3], while the various needs and solutions in the power system are extremely broad. Therefore, a broad definition with flexibility relating to the power systems general ability to manage changes, as proposed by [1], has a value in the sense of widening the understanding of the flexibility as a concept and preventing limited views on solutions.
2.2. Defining Resilience in the power system context
Resilience of the power system reflect the impact of severe events. It is a way of describing the power systems ability to deal with extraordinary disturbances, high-impact low-probability (HILP) events, or rapidly changing external conditions. Assessment of resilience include the system's ability to withstand an event, the rapid recovery from a disturbance, as well as its adaptability to prepare against future threats.
Some of the areas threatening the functionality of the power system relate to instability, cyber security, and climate change. The energy sector faces multiple threats from climate change, in particular from extreme weather events and increasing stress on water resources. Greater resilience to climate change impacts will be essential for the technical viability of the energy sector and its ability to cost-effectively meet the rising energy demands driven by global economic and population growth and electrification to decrease the carbon footprint of various sectors. Moreover, in the era of digitalization, cybersecurity is playing an increasingly important role and hacker attacks may have significant and extensive impact on the system. Other antagonistic threats to the power system involve e.g., damaging of physical assets, seizing of sensitive information, strategic investments, and control of critical delivery chains.
Resilience has also been defined in many ways, with a recent definition from CIGRE being:
- Power system Resilience is the ability to limit the extent, severity and duration of system degradation following an extreme event [4].
As described in [4] and illustrated in [5], a set of key measures can be deployed before, during, and after an event (as illustrated in Figure 2), to achieve or enhance resilience:
- Anticipation and preparation involve measures to be taken in advance, before any occurrence of an event. This may include scenario based mitigating strategies and plans for emergency actions.
- Absorption relates to the system’s ability to absorb/withstand the impact during an event, aiming for decreased or avoided consequences.
- Sustainment of critical system operations and rapid recovery relate to processes during and after an event, to maintain the available operational capabilities and to rebuild the system to be able to return to normal operation.
- Adaptation are measures taken after an event, where application of lessons learnt are basis to improve the system and increase its resilience to other events.
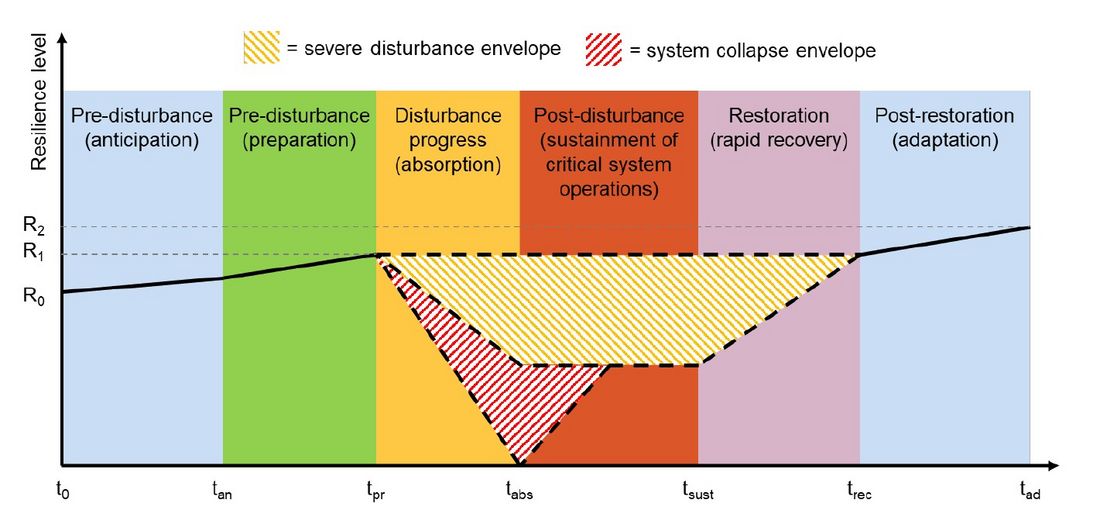
Figure 2 - The resilience trapezoid relating resilience enhancing measures to the timeframes of the occurrence of an event, from [5]
The high level of details included in and surrounding several definitions of resilience, together with the fact that parts of the definitions involve relations to solutions to increase resilience, are preventing a common definition. Resilience metrics vary as well, being e.g., measures of (possible) impact or quantities required to keep impact at a certain level. Therefore, the logic of a broad definition – with resilience relating solely to the impact of severe events – would simplify the understanding and the discussion on solutions providing increased resilience.
2.3. Resilience against extraordinary events
When addressing resilience, it is required to go into details of extraordinary disturbances threatening the power system. These may be categorized based on their main cause and impact on the power system, distinguishing three main categories: [6]
Power system-initiated events: where main cause originate from technical or operational failures inside the power system (e.g., component malfunction, faulty protective actions, operator errors, overloading), typically evolving in a cascade manner. Relatively short duration is categorizing this type of events, since, if no major mechanical breakdown occurs, restoration mainly relates to the re-energization and re-synchronisation of power system components. Power system-initiated events may in many cases be prevented by increased system reliability in the design phase, including more backup components, redundancy schemes, and parallel connections.
External events: caused by deliberate or accidental actions by a third party, e.g., cyber and physical attacks. Cyber-attacks are relatively new and are gaining in importance with the widespread digitization, big data, new technological possibilities for communication, and remote access of many devices. Cyber-attacks resulting in severe blackouts are rare, but several cyber incidents have occurred in the last years.
Natural hazard events: caused mainly by factors related to the environment, such as adverse weather (extreme wind, flooding, icing etc.), natural disasters (e.g., forest fires, landslides, earthquakes, or volcanic eruptions) or space weather (incl. geomagnetically induced currents as result of severe geomagnetic storms). Extensive tripping and destruction of power system components are often characterizing natural hazard events. Such direct impact on the mechanical structures of the power system, as well as on other infrastructures, may significantly delay the restoration process prolonging the duration of the blackout. Natural hazard events may in many cases be prevented by increased mechanical dimensioning of power system components and structures and locating them in a less exposed environment.
3. How can flexibility support power grid resilience?
In the evolving power system, novel resilience solutions are spreading out while some conventional ones are fading away, but at the same time the system gets exposed to increased stress. Figure 3 illustrates this shift, where the evolution results in a need for novel solutions providing resilience and their connection to the different timeframes of resilience enhancing measures.
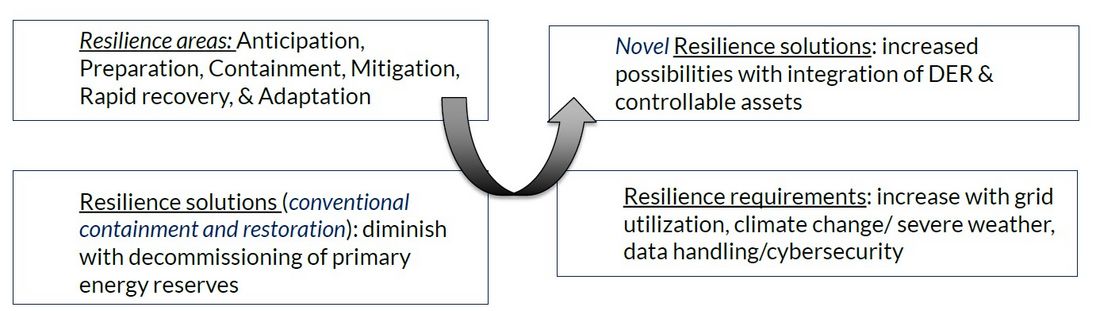
Figure 3 - Resilience solution and requirements
As solutions for flexibility emerge and are widely deployed from a variety of new devices and processes, it is worth to make a conceptual effort in order to extend their use to face reliability & security threats, i.e., to increase resilience with little or no extra asset cost for the system.
3.1. Next level of Flexibility
All power systems have an intrinsic degree of flexibility to continuously adapt generation to the existing demand; in the future, it will have to be mostly the demand (plus storage options) to adapt to the variable generation. Volatility and uncertainty are not new to power systems as the load varies significantly over time and energy sources can fail unexpectedly. Conversely, vRES can temporarily make it difficult to achieve the system balance. Both wind and solar energy sources vary considerably over hours and days, sometimes in a predictable manner, but the forecasting of their generation capacity has unavoidable limitations.
Symptoms of insufficient flexibility in the power system can include:
- difficulties in balancing supply and demand, causing frequency dynamic beyond the technical allowed range, or the need to reduce load through load shedding;
- significant curtailment in the supply of energy from renewable sources, due to excessive supply together with transfer constraints;
- deviations from the scheduled power balance of an area (and therefore also in the neighbouring areas), indicating the non-fulfilment of the balancing responsibility;
- negative energy prices, due to insufficient ability to reduce production and increase demand, together with transfer and storage constraints;
- price volatility, due to transfer constraints together with limited availability of peak production units, demand response and storage options.
A multi-faceted assessment of the available flexibility will provide information for determining investment priorities for increasing generation and transmission capacity. Utilising robust indicators may support operators to make informed decisions regarding both the optimal investment portfolio and the operational activities of managing the system.
The next level of flexibility is for flexibility solutions to become fully deployed and utilised for operation and planning of the power system, integrated in procedures for long-term planning as well as in tools for operational support, including frequency or voltage stability support. Development of solutions providing the next level of flexibility should become a hot topic in the power system sector. However, integrating flexibility solutions in the long-term planning, as well as integrating dependencies of flexibility solutions to provide stability supporting actions, involves significant risks, volatility, and uncertainty.
3.2. Risk management and energy transition
Uncertainty can be categorized as randomness or “known unknowns” and lack of knowledge or “unknown unknowns” [7]. Randomness is an independent feature associated with the nature of the uncertainty whereas lack of knowledge can be improved by knowledge sharing, data transfer, and detailed analysis. The uncertainty in input data can propagate into the design and investment decisions and affect the outcomes. A countermeasure is to analyse and adopt no-regret solutions, when they exist, implying non-negative outcomes from different possible scenarios. The risk and uncertainty become important due to the need to maintain system resiliency against events while ensuring reliability. The occurrences of extraordinary events in power systems are difficult to be predicted accurately, but the probabilities of occurrences may be estimated with a some heuristic probabilistic methods.
A system becomes resilient from proper risk-management practices. The risk management practices are required to be a structured and comprehensive with cause-consequence analysis. Prevention and control strategies in the cause-consequence analysis are designed to limit the intensity, extent, and subsequent consequences of extraordinary events. The risk management strategy is broadly classified into Stress avoidance, Stress resistance and Strain adjustment [8]. The strain adjustment is basically a mitigation strategy and deals with flexibility of the system to adapt to stress. Flexible design helps in achieving the objectives as it factors uncertainty and variability in power flow.
Power systems are traditionally designed based on the N-1 criterion, to be applied also during planned outages, so achieving a high degree of reliability with a simple stochastic approach. The flexible operation in generation, transmission, distribution, and consumption provides extra means to abide N-1 without necessarily adding new grid assets; in other terms, the flexibility mechanisms can reduce asset redundancy and therefore costs and tariffs to reach the same or higher level of secure operation, both in ordinary contingencies (reliability) and in HILP events (High Impact Low Probability). The flexibility in generation is supporting by providing fast ramping and wide ranges of operation. The transmission is becoming more flexible owing to the augmentation in HVDC and FACTS devices, allowing for enhanced control of the power flow as well as utilisation of power lines real time rating. The distribution side flexibility may utilise Distribution Management Systems to its advantages. The capability of shifting of loads from one feeder to other is a resilience based flexible approach. Demand Response also adds to flexibility and resiliency. The flexible design could help in adopting to emergency conditions, where these features act as a reserve in the hands of the system operator and in case of emergency scenarios can be easily put in operation. As long as sector coupling takes place, demand side can exploit also new flexibility sources (such as EV batteries, thermal inertia of buildings, multi-fuel District Heating, various process storage capabilities, hydrogen, etc.) to de-stress the electric system during the most acute phase of the disturbance.
To prevent damage from an extraordinary event, an accurate understanding of the possible threats from that event is required. This is obtained through the use of models to analyse and update the design basis threats such as earthquakes, tsunamis, and hurricanes. Similarly, advances in the reliability of emergency generating units and efficient power usage during a prolonged loss of supply are also important to prevent damage from such events [9]. Due to the nature of the power system, outages resulting from failures in the transmission system are less frequent than those due to failure in the distribution grids, but their failure affects larger areas, and outage durations may be much longer (for cables, especially submarine). This fact, combined with the high cost per transmission equipment require greater attention. In comparison to generation, transmission assets are extended to large geographical areas. The transmission lines spans are also more exposed when it comes to thunderstorms/cyclones etc. The following practices are important to enhance the resiliency of transmission systems:
- Underground cables: transmission lines are prone to vegetation faults, cyclones, lightning strikes etc. Underground cables at selective locations may improve resiliency. It should however be noted that underground cable faults may have significant duration.
- Periodic line maintenance: standard practices of line maintenance help in avoiding unnecessary outage due to low clearances on account of vegetation or any other reason.
- Spare availability: can reduce the downtimes significantly, but they come at a clear cost; smart asset management techniques have been developed and deployed in heavily asset-based companies.
- Digitalised and automatic maintenance procedures, based on advanced database of personnel and material: An up-to-date database of available resources for timely mobilization during crisis situations.
From the energy transition point of view, secure utilisation of existing infrastructure is imperative to maintain high levels of resilience and to ensure security and quality of supply of the systems. In parallel with conventional solutions, alternative grid development solutions, also called non-wire alternatives, emerge which use non-traditional solutions such as advanced monitoring and control of distributed energy resources and demand to defer or replace the need for specific infrastructure upgrades.
3.3. System operation
In terms of power system management, flexibility has been developed for grid operation with high variable RES penetration. System operators are primarily focused on security and reliability standards based on meeting peak demand, but this approach does not fully follow the variable and uncertain nature of the network utilisation especially due to the increased penetration of distributed energy resources and the growing electrification of the economy. Indeed, the points-in-time most stressful for the system shall no longer be limited to the highest peak demand (for active power), nor to the lowest (for reactive power), rather they will happen when the combination of variable generation and variable load gets to a minimum/maximum or to fast ramping up/down.
The mechanisms for European TSOs to achieve balance in transmission grids are already implemented through the existing networks codes and their subsequent implementation in the different Member States. Additionally, the Directive (EU) 2019/944 also states that “Member States shall provide the necessary regulatory framework to allow and provide incentives to distribution system operators to procure flexibility services, including congestion management in their areas”.[10] These services can materialize in the form of solutions for congestion management, as well as non-frequency ancillary services such as steady state voltage control, fast reactive current injections, inertia for local grid stability, short-circuit current, black start capability and island operation capability.
3.4. Grid planning
The design and building of new generators and transmission system takes long time and the investment planning process is the initial step to ensure that the power system will have sufficient adequacy, flexibility and resilience. In regulated scenarios, this function was carried out via a centralized planning model in which industry participants and government agencies jointly assess potential requirements for resiliency. In market-based scenario, sufficient investment signals regarding the necessary adequacy level, and the need for flexibility are required. In the absence of sufficient investment planning clarity, the power system may lack the ability to operate with sufficient resilience. The resilience is thus factored in at the long-term planning stage in several power systems. For instance, the need of black start sources are required to be planned, so that they integrate with the rest of the system. Sector coupling initiatives can become new sources of black start capabilities, exploiting the energy stored in other form (e.g., heat in buildings) or in other devices (e.g., EV), provide their interface through conversion devices is capable to handle the reverse flow. Grid planning may also consider the geography and locational aspects.
Flexibility is relevant to many aspects of the planning process [11]. Investment planning, in the form of network development plans, usually, utilise reference scenarios to identify the technical characteristics of main investments, however current and future practices shall rely on methodologies assessing technical and economic potential to be deferred or replaced by flexibility. Therefore, for each network development case, system operators will have to analyse the feasibility and cost-effectiveness of any flexibility solution versus investments in traditional infrastructures. As a result, the network development plan will highlight areas or parts of the grid where there is a potential need for flexibility services.
In this sense, implementation of the flexible investment planning is a relevant aspect to consider by system operators as an alternative to classic infrastructure development plans. When considering the potential of flexibility from a resilient perspective, it is important to develop mechanisms based on forecast changes in customer load and plan upgrades or grid extensions, and also to anticipate asset replacement needs as equipment reaches the end of its useful life.
Accordingly, some of the solutions to take into consideration when addressing needs in development plans include:
- Optimising infrastructure investments.
- Deferring or avoiding asset reinforcements.
- Efficient maintenance planning, asset replacement, and connection works.
- Quality of supply improvements.
- Reduction of network extension timescales.
- Increasing capacity of existing grid assets.
Regarding planning of lower voltage levels, it is challenging to develop long-term development plans since these to a higher extent depend on shorter economic cycles and social dynamics. Distribution system operators managing lower voltages need to improve and refine their forecasts for electricity use and distributed resources (especially storage devices) also behind-the-meter, which can substantially change the load profiles, to ensure that the capability of the distribution system is expanded in a cost-effective manner using smart solutions and intelligent asset development, and, at the same time, helping to proactively determine the development of the distribution system at higher voltage levels.
Investment planning activities are also significantly affected by RES variability, which becomes more complex and affected by a high level of uncertainty. Grid investments are capital intensive and infrastructures lifetime spans over several decades. Due to widespread RES and DER deployment, the generation and load scenarios upon which the cost-benefit analyses for new grid infrastructures are based are continuously and rapidly changing. As a consequence, when a new line is commissioned, the technical-economic benefits it was initially supposed to reap could prove significantly different than expected. Additionally, building new lines meets more and more public opposition, which makes planning activities even longer and affected by uncertainties. Variable flows from RES are generating a new type of intermittent congestion which can sometimes be better compensated by resorting to system flexibility: in many cases, an investment in a new line/cable would not be economically justified. Thus, establishing new T&D grid planning methodologies, considering e.g., the opportunity to install storage devices and other flexible alternatives to building new lines, is an important step forward. With local solutions to cope with RES generation peaks, the congestion in the grid can be reduced in and less environment-impacting manners (though not necessarily less expensive). At the same time, the increase of flexibility available in the network will positively contribute to its resilience.
4. Conclusions
Power system resilience reflect the impact of severe events and is an overarching concept, covering the whole spectrum of the power system from design and investment decisions to planning, operations, maintenance, and asset management functions. As such, the concept of power system resilience applies to the planning time frame that looks to build resilience into the future network, as well as the operational time frame, in which security is managed by optimizing the inherent resilience of the existing power system.
Flexibility concerns the power systems ability to manage changes, with flexibility features able to improve the resilience characteristics of the broader view system of systems, provided that they are integrated in grid planning, in defence plans, and properly evaluated in the energy market design. Flexibility capabilities need to be considered from the planning stage, using a holistic approach aimed at grids to be flexible and resilient by design. Flexibility resources can also facilitate the restoration process by exploiting distributed black start capabilities including sector-coupling, which adds a new dimension to the necessary interactions pattern between electrical TSOs and DSOs, with utilities from other sectors. Power system planning for the future grid must embrace a wide range of network and non-network options to create operational flexibility options, including more active demand management techniques and customer-sensitive smart load shedding procedures.
The next level of flexibility is seen as being fully deployed and utilised for operation and planning of the power system, being integrated in procedures for long-term planning as well as in tools for stability support. The integrated dependency of flexibility directly impacts the resiliency of the power system, thus flexibility solutions intended to provide resilience support must be rigid and secure to provide the trust required for operation and planning.
Many of the worldwide ongoing initiatives can provide highly relevant knowledge to the question of How can flexibility support resilience? Indeed, they show the relevance and the potential values to be unlocked, with potentially some low hanging fruits to start with. Some of the examined areas include:
System Integrity Protection Schemes (SIPS) are solutions found in power systems around the world which are used to mitigate large disturbances. Flexibility solutions, such as fast control of load and distributed generation, can be used to improving existing SIPS and enable development of new solutions, including: Controlled load-shedding, Islanding and island operation, Distributed recovery & black-start, and Emergency controls.
System Technical Performance can be improved through the use of flexibility solutions, where the resilience of the grid is increased. Flexibility solutions such as enabling of flexible transfer capacities and controllability of distributed assets support the resilience in the sense of: increased the number of mitigating measures based on the vast amount of controllable assets; improved performance regarding prevention of congestion, distributed voltage support, enhanced stability and reduced system losses; simplified maintenance planning as well as operational processes.
Alternative Grid Development, provide an agile and sustainable development of the power grid and can support power system resilience through: optimising the investment levels versus the operational costs, and secure solutions to defer investments enabling more efficient planning processes. With a regulation moving towards supportive of the total expenditure, alternative grid development solutions can become reality as both short- and long-term resilience enhancement solutions.
The economic value provided by large scale flexibility solutions, including reduced costs for security measures (e.g., redispatch), can increase the benefit of maintaining high levels of resilience and thus provide incentives for resilience-enhancing investments. Additionally, cyber security is an area with increased focus, where common platforms for data sharing and AI & machine learning solutions are part of the digitalisation of the power system. Finally, standardisation of solutions (architecture, tools, & platforms) is important to increase the reliability & acceptance in order for large scale deployment of flexibility.
Acknowledgements
This paper is the result of a Task force between ETIP SNET WG 1 on Reliable, economic, and efficient energy system and ISGAN Annex 6 on Power Transmission & Distribution Systems. The final report of the Task force, [12], provides the full details of this work presenting a global view on how flexibility can provide value for the resilience of the power system. A total of 15 experts, from 12 countries in Europe and Asia, have been contributing to this work and their efforts are gratefully acknowledged.
References
- E. Hillberg et.al., “Flexibility needs in the future power system”, ISGAN, 2019, DOI: 10.13140/RG.2.2.22580.71047
- CIGRE Working Group 37.10, "Methods for planning under uncertainty – towards flexibility in power system development", Electra, vol. 161, pp. 143-163, 1995
- CIGRE Working Group C5.27, “Short-term flexibility in power systems: drivers and solutions”, TB 800, 2020
- CIGRE Working Group C4.47, “Defining power system resilience”, ELECTRA no. 306, 2019
- CIGRE Working Group C4.47, “Resilience of interdependent critical infrastructure”, ELECTRA no. 320, 2022
- E. Hillberg, “Perception, Prediction & Prevention of Extraordinary Events in the Power System”, Doctoral thesis, NTNU, 2016
- T.Hardy, M. Knight, A. Veeramany, & J. Woodward, “Resilience Metric Formulation Considering Transactive Systems”, Pacific Northwest National Laboratory, 2018
- S Widergren, A Shankar, B Kelley, J Taft, R Melton; “Toward a Practical Theory of Grid Resilience A Grid Architectural Approach”, April 2018, Northwest National Laboratory
- Electric Power Research Institute, “Electric Power System Flexibility: Challenges & Opportunities”, 2016
- European Parliament and the Council of the European Union, Article 32 (3), Directive (EU) 2019/944 of the European Parliament and of the Council of 5 June 2019 on common rules for the internal market for electricity and amending Directive 2012/27/EU
- J. Cochran, et al, “Flexibility in 21st Century Power Systems”, National Renewable Energy Laboratory, 2014
- European Commission, Directorate-General for Energy, “Flexibility for resilience: how can flexibility support power grids resilience?”, editors: I. Oleinikova, A. Iliceto, E. Hillberg, Publications Office of the European Union, 2022, ISBN 978-92-76-52781-7
