High Voltage Direct Current transmission line and human health
Authors
M. PLANTE M.D., Hydro-Québec, Canada
M.B. BARBIERI Eng., Universitad national de la Plata, Argentina
J.A. BULCAO M.D., Medical Advisor, Brazil
P.A. CABANES M.D., EDF, France
F. DESCHAMPS Eng., RTE, France
S. JACKSON M.D., EDF-Energy, United Kingdom
L. KORPINEN Dr. Tech., M.D., Elenhe Ky, Finland
I. MAGNE Ph.D., EDF, France
S. NAKASONO Ph.D., Central Research Institute of Electric Power Industry, Japan
D.H. NGUYEN Eng., Hydro-Québec, Canada
G. OSTIGUY M.D., Hydro-Québec, Canada
D. STUNDER Ph.D., Amprion GmbH, Germany
H. TRIPP Ph.D., National Grid, United Kingdom

Summary
Overhead HVDC lines high static electric fields and the associated ion currents can produce superficial sensory sensations of hair and skin. The physical effects are limited to the surface of the skin and the hair and preclude any direct effect on internal physiology. In general, electric field levels measured under existing lines up to 600 kV suggest that the probability for a person to experience some subtle sensory perception is low. These effects do not constitute an adverse health effect. No scientifically robust evidence suggests that this exposure is associated with any human health effects. Magnetic field levels at ground level are low and similar to the natural magnetic field levels. Exposure to both magnetic and electric fields under an HVDC line will comply with limits put in place to protect members of the public.
In the case of a single underground HVDC cable, such as when the two cables of a bipolar line are far from each other, magnetic field levels at ground level can be several times higher than the natural field but almost always lower than the recommended limit to avoid interference with cardiac implants. Nevertheless, this particular situation should be paid attention to when designing an underground HVDC line.
Keywords
Electric field, Health, HVDC transmission line, magnetic field, static field1. Introduction
The possible health effects of electric and magnetic fields produced by high voltage alternating current power lines have been the subject of a large international research effort over the last 40 years. Overall, the results have been reassuring. No particular health effect has been reported as likely to occur at field levels below the current exposure limits [1].
Few concerns have been expressed about the possible effects of static electric and magnetic fields produced by high-voltage DC power lines (HVDC) and few studies have been conducted on the subject. However, new DC power lines are routinely planned and commissioned and are subject to the environmental assessment process, which includes a human health risk assessment. This article summarizes the relevant characteristics of HVDC power lines to human exposure and explains why the particular electric and magnetic environment around a DC power line is not expected to have any impact on human health.
The electric and magnetic fields (EMFs) produced by a DC line are static, while those produced by an alternating-current (AC) line alternating, i.e., their strength varies at a frequency of 50 or 60 cycles per second. This distinction is important concerning its effects on the human body. Contrary to HVAC lines, HVDC lines do not induce any currents and voltages within the body and there is no known mechanism of interaction at typical field levels found under HVDC with internal organs. The following text only covers static fields and air ions produced by HVDC lines.
2. Static magnetic fields
2.1. Static magnetic field values close to HVDC transmission lines
The unit of measurement of magnetic fields is amperes per meter (A/m) but the tesla (T), which is the unit of magnetic flux density is generally used to express the magnetic field strength.
The Earth is surrounded by a permanent static magnetic field, which can be detected with a compass. In that field, all humans are exposed to ranges in strength from 35 microteslas (μT) at the Equator to 70 μT in polar regions. It is a static field pointing toward the magnetic north with a constant strength at a given location.
The magnetic field produced by an HVDC line is proportional to the current flowing in the line but also depends on the height of the conductors above ground, their spatial disposition, and the operating mode (monopolar or bipolar). Typical values for an overhead HVDC vary from a few microteslas to a few tens of microteslas, measured one meter above ground. Values may be somewhat higher for an underground HVDC line but would rarely reach 100 μT, one meter above ground. Figure 1 shows the magnetic field profile produced by a 320 kV overhead line carrying 2000 A, excluding the Earth’s natural magnetic field. The field decreases rapidly with distance, reaching a maximum value of 10 μT at the middle of the line, while it is 4 μT at the edge of the right-of-way (ROW).
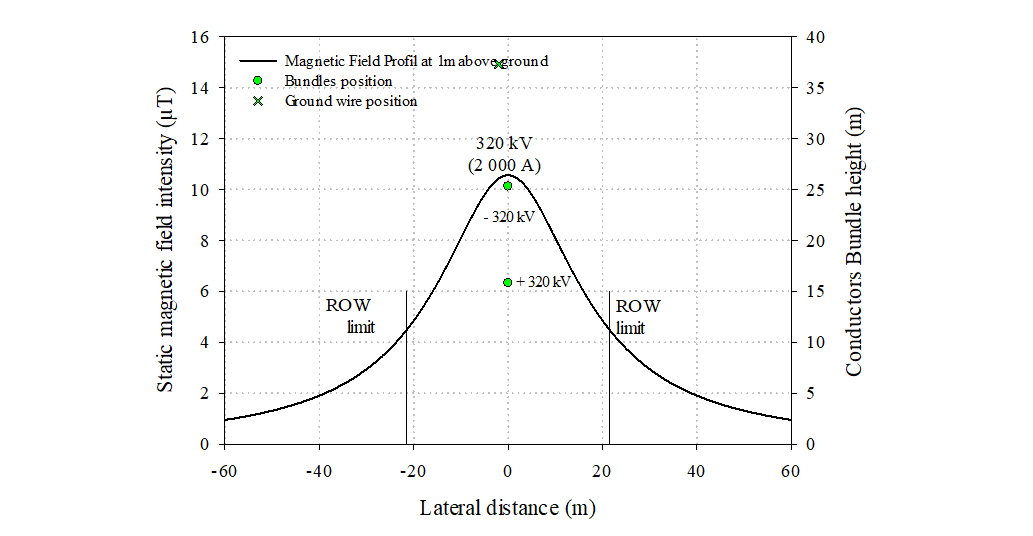
Figure 1 - Magnetic field profile for a 320 kV DC line carrying 2000 A
The magnetic field profiles above were calculated ignoring the Earth’s magnetic field. In reality, the ground-level field beneath the DC line will be the vector sum of the Earth’s magnetic field and the field produced by the line. The total magnetic field is higher than the natural field when both components point in the same direction and lower when they are in opposite directions. The HVDC line used as an example runs at an angle of 129 degrees with the magnetic north, and the natural magnetic field strength at this location is 53 μT. The profile of the total magnetic field is shown in Figure 2.
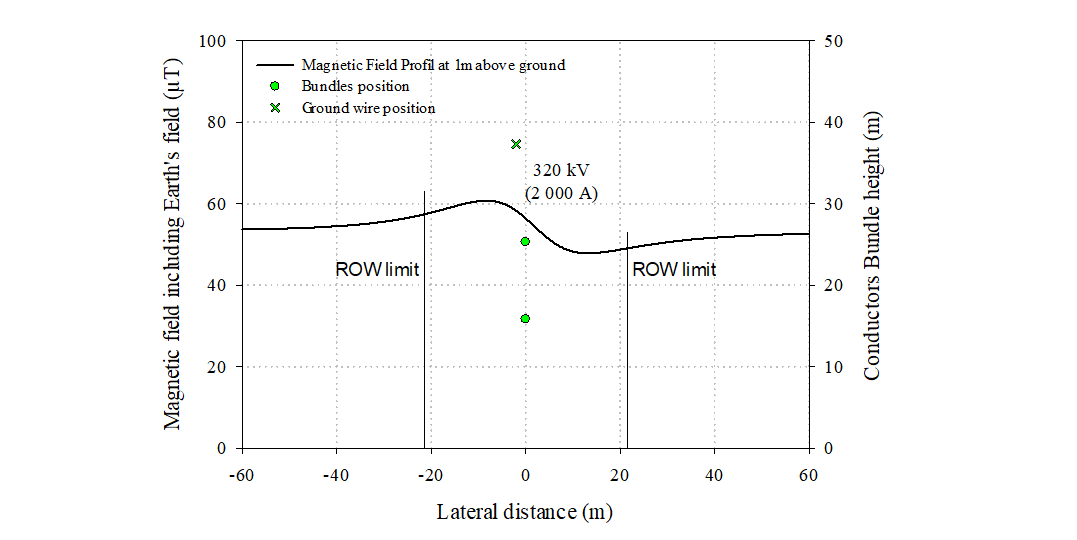
Figure 2 - Total magnetic field under the 320 kV which runs with an angle of 129 degrees with the magnetic north
The total magnetic field increases from 53 to 61 µT on the left side of the line and decreases from 53 to 47 μT on the other side. The distortion diminishes with distance and becomes negligible at approximately 50 meters from the edge of the right-of-way. In conclusion, the DC line’s area of influence on the Earth’s magnetic field extends up to approximately 50 m from the centerline.
Although the amplitude of the distortion is moderate, it may be significantly higher or lower depending on the local field, the angle between the 2 vectors, and the nature of the line.
Figure 3 shows the profiles of a similar line but with conductors placed in a horizontal configuration. The resulting magnetic field is much less distorted.
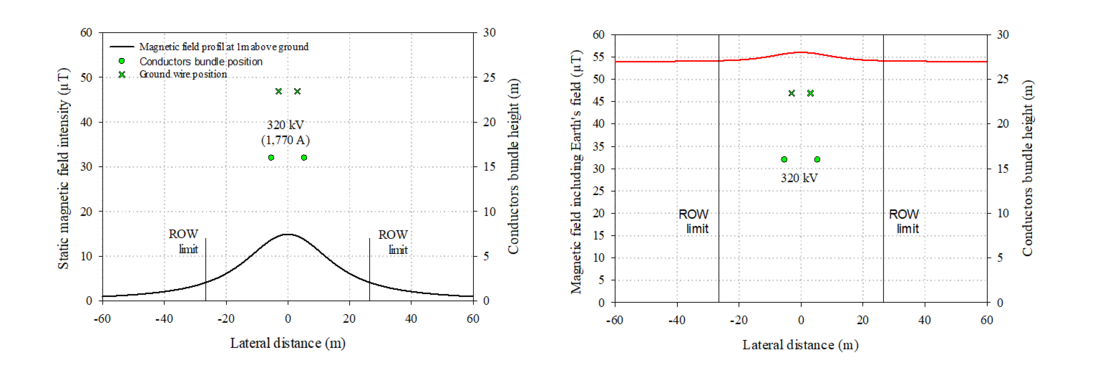
Figure 3 - Magnetic field profile without considering the natural field (left) and the total magnetic field (right)
The magnetic field profile of an underground line differs from that of an overhead line: The maximum field at 1 meter above the ground is usually higher, and the field decreases more quickly laterally so that the area of influence of the underground line is less wide and the magnetic field is higher near the ground due to the proximity of the cables.
Figure 4 shows the magnetic field profile for an underground 400 kV DC line, buried at 1.31 m. Profiles are shown at the height of 1 m above ground (usual height of measurement [2]) and also at the height of 10 cm (worst case scenario of a person with a cardiac implant lying on the ground, see below). The distortion of the natural geomagnetic field is limited to a few meters from the centerline.
In the case of a single underground HVDC cable (such as when the two cables of a bipolar line are far from each other), magnetic field levels are given by a simple formula: B (µT) = 0.2*I/D where I is the current in Amps and D the distance to the center of the cable. As an example, with I = 1500 A and D = 1 m (burial depth) the field at ground level is 300 µT.
At ground level, the field can therefore be several times higher than the natural magnetic field but still lower than the recommended limit to avoid interference with cardiac implants (500 µT) except when the burial depth is significantly lower than usual (at least 1 m). Nevertheless, attention to this particular situation should be paid when designing an underground HVDC line.
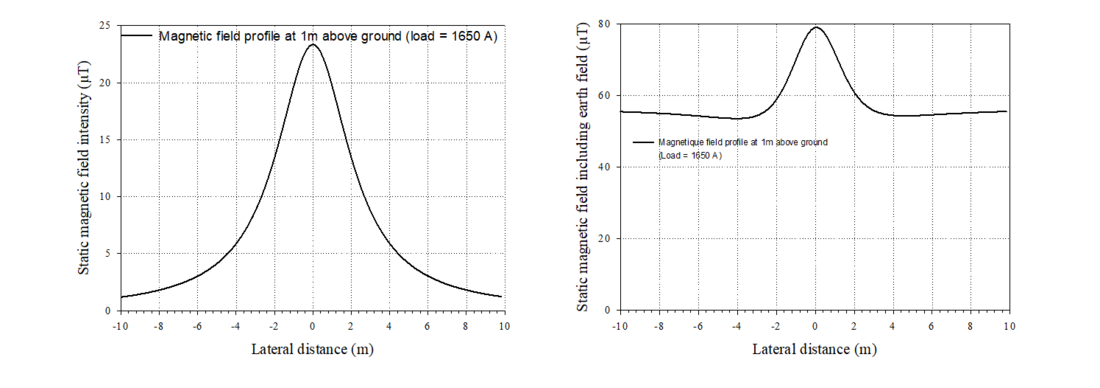
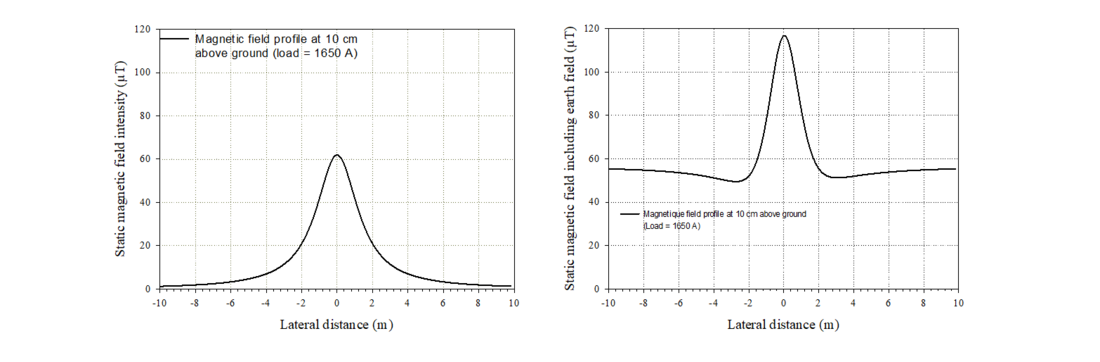
Figure 4 - Magnetic field profile without considering the natural field (left) and total magnetic field (right) for underground cables at 400 kV DC, buried at 1.31 m with a separation of 0.38 m, carrying a load of 1650 A. The upper profiles were calculated 1 m above ground level and the lower profiles were calculated 10 cm above ground level
In practice, the measurement of statics fields lower than 1 µT is difficult considering the measurement uncertainties and also the local variations of the Earth field which might be significant, particularly in urbanized areas. In practice, the maximum distance at which the influence of an HVDC line remains measurable will be lower than 50 m for overhead lines and lower than 10 m for underground lines.
2.2. Interaction mechanisms
Static magnetic fields do not induce electric fields and currents in a resting body. However, a rotational motion in a uniform field or a movement along a field gradient will induce a current during that particular movement. The velocity of the movement and the magnetic field intensity will determine the amplitude of the current. Calculations show that the rotation of the human body within the range of intensity of the natural magnetic field cannot be fast enough to induce any significant current. For example, a complete rotation of the body along the vertical axis in one second (1 Hz) in a static field of 50 µT induces the same current as a 50 Hz field of 1 µT. However, induced current can be significant during normal movement around fields greater than 1,5 to 2 T like those present near a magnetic resonance imaging device (MRI).
Static magnetic fields can produce mechanical effects on some biological objects. Magneto-orientation will produce a torque on paramagnetic molecules. Generally, these forces are considered too weak to affect biological material unless the magnetic field intensity is in the order of a few teslas. However, this mechanism is present in animal species capable of orientation and navigation during their migration. The biological mechanism of the magneto-sensitivity of some animal species is not well described nor characterized. The orientation of paramagnetic molecules or proteins is one of the accepted hypotheses but has not been demonstrated [3]. The magneto-mechanical translation is another type of interaction that can occur in the presence of gradients, producing a translation force on both diamagnetic and paramagnetic materials. The force is proportional to the magnetic flux density and its gradient. Calculations show that this mechanism requires magnetic flux densities 5 to 6 orders of magnitude greater than the intensities found in nature and around HVDC.
Finally, a static magnetic field may intervene in certain chemical reactions involving a radical pair based on the spin of the electron. An applied magnetic field can affect the recombination rate of the reaction. Although experimental evidence for such effects in biochemical systems has been reported, their biological significance is not clear at present [4].
Humans do have trace levels of magnetite in the brain and other tissues in the form of nanoparticles with a size of about 100 nm. However, their presence does not confer an ability to detect the weak geomagnetic field. Moreover, there are no indications so far that their presence causes any detectable effect under very high static magnetic fields of 2 T and more.
2.3. Static magnetic field and human health
The human body is transparent to magnetic fields since it does not naturally contain metals in a significant amount reacting with such fields. The body can be exposed to very high levels of static magnetic fields with no observed harmful effects. Medical imaging equipment (MRI) produces static magnetic fields of roughly 2 T, which is 40,000 times stronger than the Earth’s magnetic field. MRI used in research has used fields up to 8 T strong [4]. Widespread use of imaging equipment over the past three decades and research to ensure their safety has not revealed any harmful effects as long as any metallic implant reacting to magnetic fields is avoided. Experimental studies have reported minor physiological changes in fields of 2 T or more. Transient symptoms occasionally occur during magnetic resonance imaging procedures. They arise from too rapid movement of the body in the magnetic field, or the large magnetic field gradient found a few meters around the device. During such movement, a current is induced in the body and may be strong enough to lead to transient effects such as vertigo, dizziness, or a metallic taste in the mouth. By slowing such movement, the symptoms are generally avoided. Theory, experiment, and experience from hundreds of millions of MRI procedures over the past 50 years have shown that this imaging method is extremely safe [5].
2.4. Exposure guidelines
The International Commission on Non-Ionizing Radiation Protection (ICNIRP), an association formally recognized as an official collaborating non-state actor by the World Health Organization, issues recommendations on safe exposure levels for workers and the public. Those recommendations are the authoritative scientific basis to which many countries around the world refer in adopting exposure standards. The recommended values set include a safety margin and thus are not the threshold above which harmful effects may appear. The recommended limits for worker exposure are 2 T for the head and torso, and 8 T for the arms and legs. The recommended limit for the public is 0.4 T regardless of the part of the body exposed [4]. In Europe the limit for the public is 40 mT, coming from older ICNIRP guidelines [6, 7].
Another international organization, the International Committee for Electromagnetic Safety (ICES), under the oversight of the Institute of Electrical and Electronics Engineers, has developed guidelines for exposure to static magnetic fields [8]. Based on experimental studies on humans, ICES estimates that the average threshold for detectable physiological effects is about 1.5 T. Given the individual variability anticipated at that threshold and an extra safety factor of 3, the recommended exposure level for workers is 353 mT. A further safety factor of 3 is applied for the public, giving a recommended limit of 118 mT.
2.5. Implanted cardiac pacemakers and defibrillators
Static magnetic field limits that must not be exceeded may vary from one manufacturer to another. In the absence of a specific limit, it is recommended that pacemaker or defibrillator wearers not be exposed to more than 500 µT. This value of 500 µT is defined as the Action Level for active implantable medical devices (AIMD) in the 2013/35/EU directive for workers [9]. Some standards give the value of 1 mT for interference level [10].
This 500 µT field level is never reached under an HVDC transmission line, and neither over an underground line, even at ground level. Only the particular situation of single cables (such as when the two cables of a bipolar line are far from each other) buried at a low depth (much less than 1 m) could result in magnetic fields meeting the 500 µT limit. Therefore, attention to this particular situation should be paid when designing an underground HVDC line.
3. Static Electric field
3.1 Natural electric field
There is a static electric field everywhere on the surface of the earth with an intensity of about 100 V/m. The natural electric field is due to the electric charges in the upper atmosphere which is ionized by the particles of the solar wind. This results in diurnal and seasonal variations, which increase with solar storms. The intensity shows diurnal and seasonal fluctuations in a range of intensity between 50 and 300 V/m nearly 90% of the time [11]. When a thunderstorm approaches, the electric field reaches much higher values, on the order of 10 kV/m to 20 kV/m at ground level (figure 5) [12]. Generally, the earth's surface corresponds to a negative charge and the upper atmosphere corresponds to a positive charge.
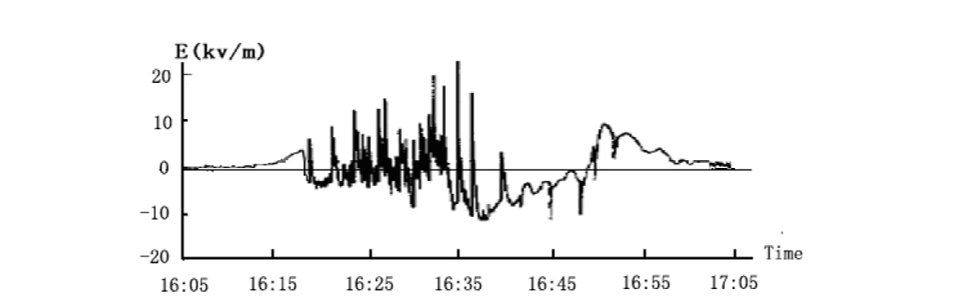
Figure 5 - Electric field strength at ground level during a storm [12]
3.2. Electric field and ion current produced by an HVDC line
The level of electric field produced by high-voltage lines depends on several factors including the voltage of the line, the height of the conductors above the ground, the spatial configuration of the conductors, and atmospheric conditions. The electric field reaches a few kV/m under the conductors. It decreases rapidly with distance and generally reaches a value close to the natural level within a hundred meters from the center of the line.
Figure 6 shows a typical electric field profile calculated for a 320 kV line with conductors in a horizontal configuration. The electrostatic field is calculated with no corona effect.
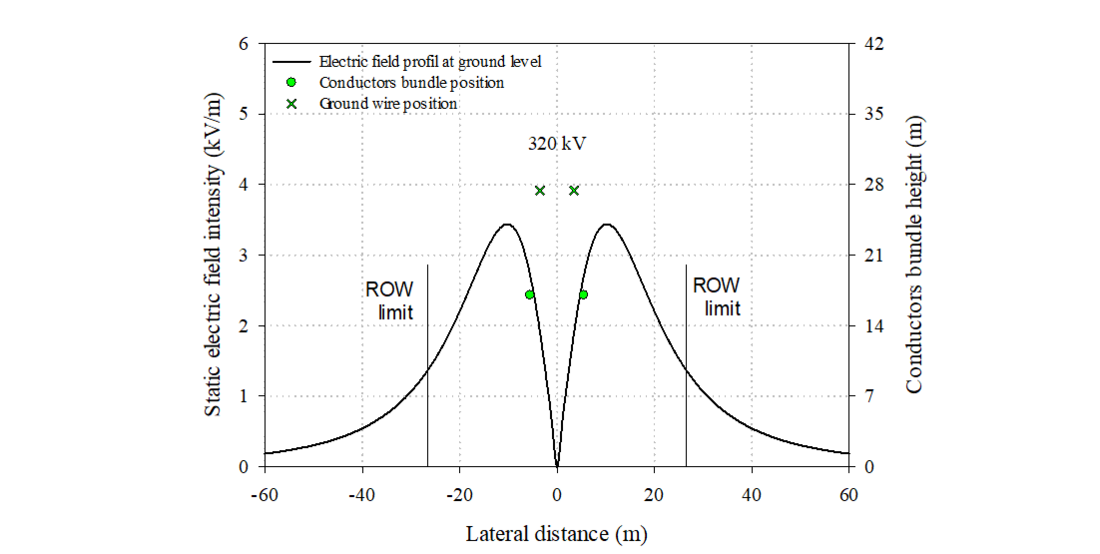
Figure 6 - Electric field profile of a 320 kV line with conductors placed in horizontal configuration
3.3. Air ions and ionic current
When the electric field level at the surface of the conductors exceeds a threshold value (2800 kV/m for dry air), spontaneous micro-discharges at the surface of the conductors occur, called corona discharges. These discharges ionize the air molecules: each ionization produces a negative and a positive charge. Although alternating-current transmission lines produce air ions, most of them are attracted back to the conductor and neutralized with each alternating cycle. In the case of an HVDC line, ions of opposite polarity to the conductor are immediately attracted by the electrostatic force, and the ion of the same polarity is repelled by following the electric field lines and the wind.
Air ions are a natural phenomenon generated by various atmospheric and natural events. Air ion concentrations near ground level vary over a wide range. Fair weather values over land are typically in the range of 500 to 1000 ions/cm3 but can easily vary from 200 to 3000 ions/cm3 of both polarities [13]. Air ion concentrations can reach levels over 1,000,000 ions/ cm3 near some sources. Figure 7 shows examples of the total density of air ions measured in a variety of locations et sources [14].
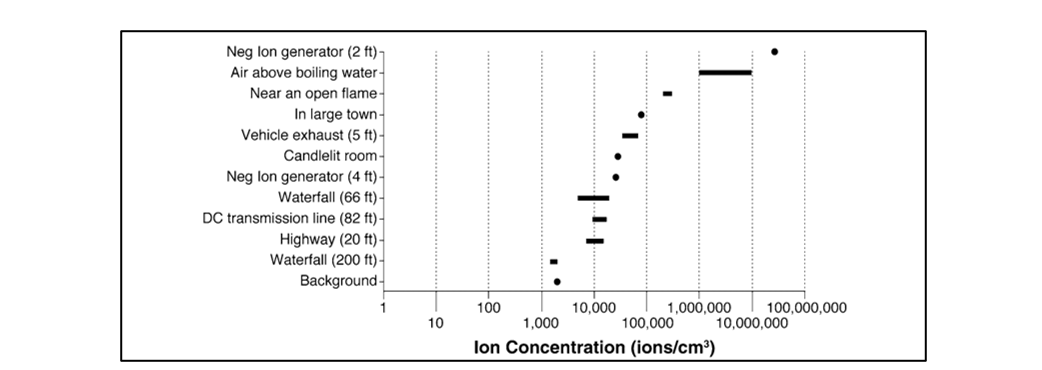
Figure 7 - Air ion concentrations in a variety of locations and near sources [14]
Reported values of air ions concentration under an HVDC line vary according to the intensity of the corona discharges, and atmospheric and wind conditions. Air ion concentrations are typically below 10,000 ions per cm3 and rarely exceed a few tens of thousands of ions per cm3.
Air ions increase the electric field level already present under the transmission line (figure 8) and the displacement of ions from the conductors to the ground produces a current that can be measured at the ground by appropriate devices, expressed in current density unit (A/m²).
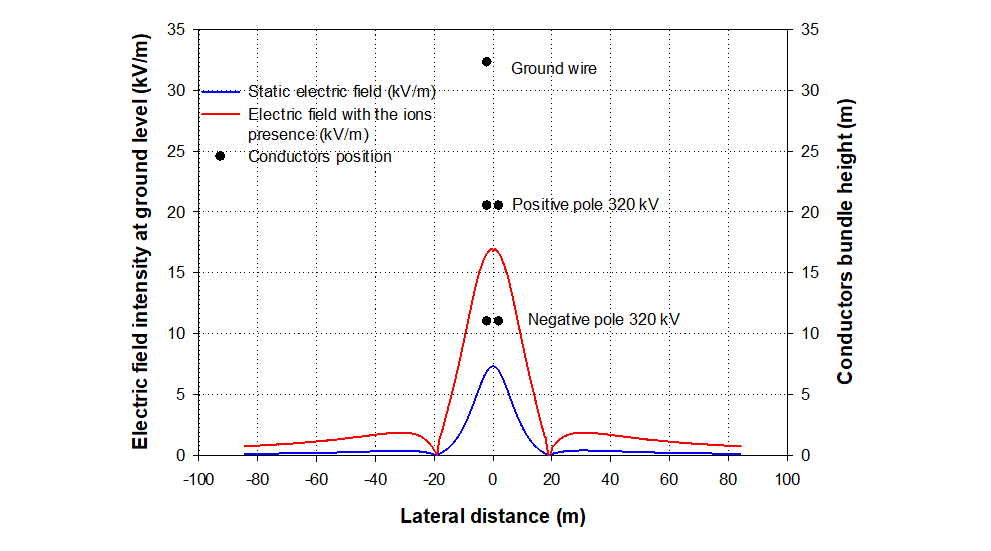
Figure 8 - Electric field profile, with and without the presence of air ions
Current density varies constantly according to the intensity of the corona effect and the atmospheric conditions. Depending on the wind, the ions can move laterally over several tens of meters and can be detected up to a few hundred meters. The negative ions may end up under the positive pole. Ion concentrations and current density values under the negative conductor are generally higher than those under the positive conductor.
The data collected during a long-term measurements study undertaken under a 450 kV DC line in Canada [15] over 20 months have shown that the highest average ionic current was 3,6 nanoamperes per square meter (nA/m²) 7.5 m away from the centerline for the positive side of the line and – 7.3 nA/m² 7.5 meters away from the centerline for the negative side of the line. The average value at the edge of the right-of-way was 1.1 nA/m² on the positive side and -4.5 nA/m² on the negative side. The current density was less than 39.3 nA/m² during 99% of the time. The results were comparable to the previous data obtained under an experimental 450 kV HVDC line over about one year. Current densities measured under other existing lines up to 600 kV have reported average values of a few tens of nA/m² [16]. In theory, higher current densities are expected with higher corona losses possibly occurring at higher voltages. An experimental study carried out over 18 months with a bipolar 900 kV HVDC line has shown a median value of about 250 nA/m² and extreme values up to 600 nA/m², measured directly under the positive conductor. At 30 meters away from the conductor, values were about a few tens of nA/m² [17].
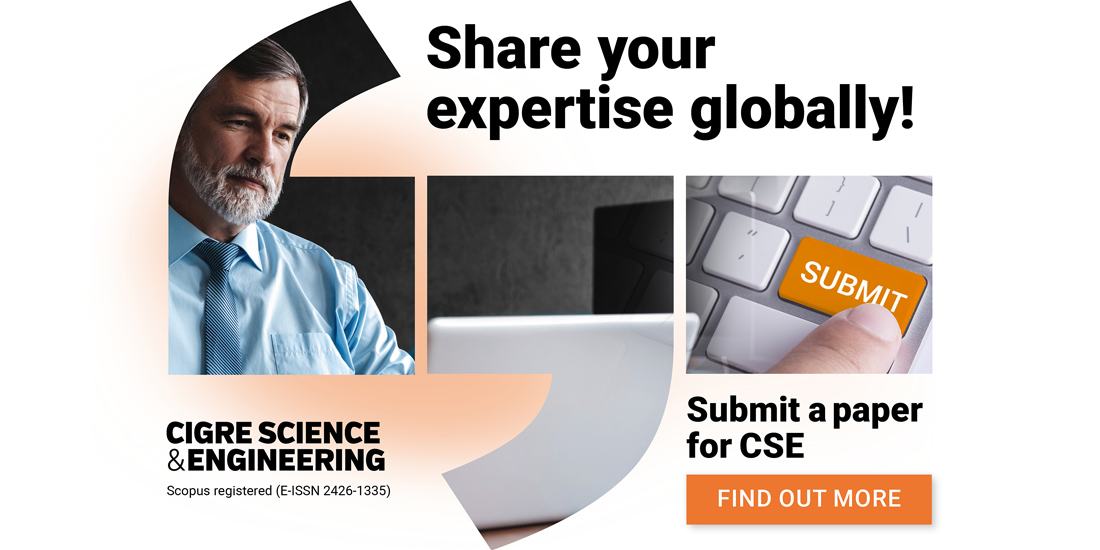
3.4. The effect of static electric field in humans
There are no known harmful effects from exposure to the static electric field. When a person's body is exposed to a static field and the person is well grounded (for example, bare feet on a wet floor), the body cannot accumulate electric charges and remains at the same electric potential as the floor. If this person is well insulated from the ground (for example, shoes with insulating soles), electric charges can accumulate and cause a rise in the electric potential of the body. The phenomenon is slow because it is the electric charges present in minute quantities in the air that allow this accumulation. The process is slow and very different from what happens under an AC transmission line where charges are immediately acquired by induced current through capacitive coupling (figure 9).
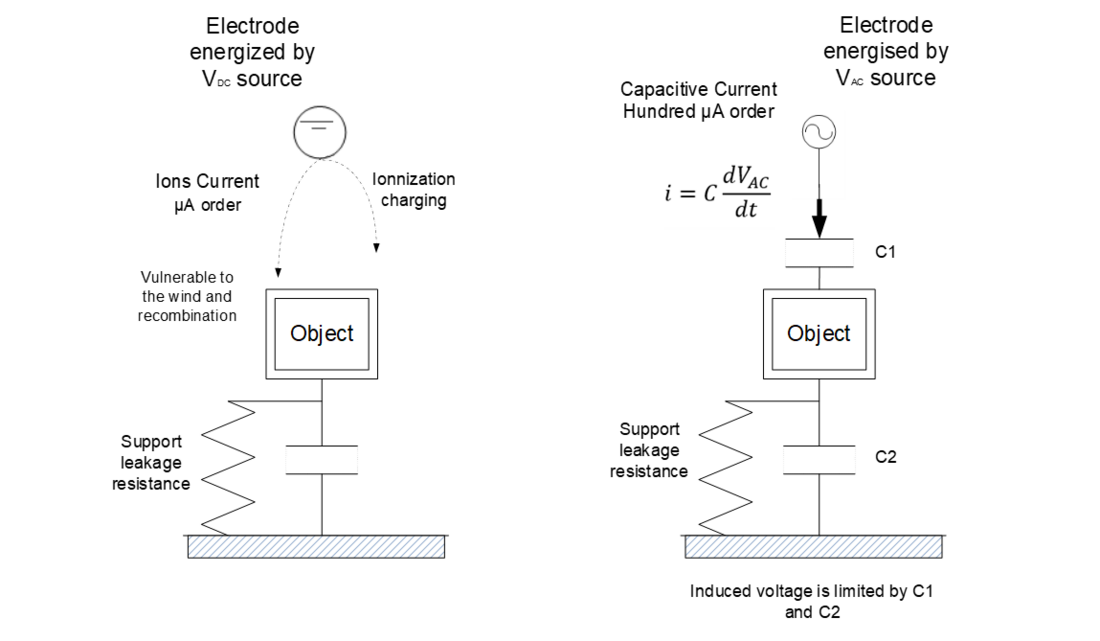
Figure 9 - voltage build-up of an insulated object under HVAC and HVDC
Due to the electrostatic repulsion between electric charges of the same polarity, the accumulation of charges is strictly confined to the surface of the skin and hair. Under an HVDC line, the electric field inside the body is zero. For this reason, it is possible to subject the human body to an electrical potential of several hundred thousand volts without any harmful effect. If the accumulated charges are sufficient, the hair will tend to stand up and repel each other. In science museums, it is common to invite the public to undergo this experiment with the help of a Van de Graff-type charge generator for the pleasure of seeing the hairs stand up on their heads as the potential increases. The experiment is safe.
If the electric potential of the body exceeds the value of 500 V, the person will experience a small electric shock when touching a grounded object. The opposite phenomenon can occur when a properly grounded person comes into contact with a conductive object that is electrically isolated from the ground and is charged. Spark discharges are regularly reported under HVAC transmission lines but are unlikely to occur under HVDC lines, due to the slow charging of the body [18]. If it does occur, the phenomenon may be surprising but is harmless.
There have been relatively few experimental studies on the effects of static electric fields in humans. Contrary to an alternating electric field, the interaction of a static electric field with the human body is limited to the surface of the skin and physical considerations preclude the possibility of any direct effect on internal physiology. A systematic review of the biological effects of exposure to static electric fields in humans and vertebrates was published in 2017 [19]. The review did not find evidence that static electric fields have adverse biological effects on humans and animals. There was clear evidence that humans and animals can perceive the presence of a static electric field at sufficiently high levels. The data strongly supports the role of superficial sensory stimulation of hair and skin as the basis for perception of the field as well as reported indirect behavioral and physiological responses.
There is also good evidence showing that the perception threshold can be lowered in the presence of air ions which increase the nominal electric field. Two experimental studies on human subjects have addressed this question.
Human perception of the static electric field and ion currents was studied in 48 subjects in a specialized exposure facility allowing whole-body exposure [20]. The study reported an average static electric field perception threshold of 45 kV/m. The electric field was only perceived at the level of the skin: the subjects described a discrete tactile sensation, felt in most subjects at the level of the scalp but also the level of the skin of the face. Most of the subjects judged the sensation as neutral and no subject described the sensation as painful. In this study, there was no statistically significant correlation between the subjects' detection threshold and the abundance of hair or hair characteristics. The subjects were also subjected to two ion current levels: 60 nA/m² and 120 nA/m². The results showed that for most subjects, the 60 nA/ m² current did not affect the electric field detection threshold. However, a current density of 120 nA/m² showed a decrease in detection threshold from 45.1 kV/m to 36.9 kV/m.
A larger study using a similar approach compared the detection thresholds of static electric field, 50 Hz alternating electrical field with or without the presence of an ion current [21]. A total of 203 participants were exposed to various conditions using a double-blind experimental setting. Four levels of electric field were tested up to 38 kV/m with or without their corresponding ion current densities up to 400 nA/m². Compared to the previous study, the detection thresholds in this study were 18.69 kV/m without an ion current and 18.22 kV/m with an ion current of 80 nA/m². However, at higher field levels, the presence of a higher ion current decreased slightly the perception threshold. The percentage of participants capable of perceiving the field increased from about 30% to 90 % from 14 kV/m to 38 kV/m. A remarkable finding was shown that simultaneous exposure to a 4 kV/m alternating electric field decreases significantly the perception threshold to 6.76 kV/m. Close to 40% of participants were able to perceive a 2 kV/m static field in the presence of a 4 kV/m alternating field. The study results have also demonstrated that static electric fields are easier to perceive under high relative humidity (50%) than low relative humidity (30%).
3.5. The effect of air ions on the human body
From a toxicological or physical perspective, there is no scientific basis to suspect that air ions would have significant physiological or pathological effects.
From a toxicological perspective, a concentration of 10,000 ions/cm3 is extremely small as it translates to a concentration of approximately 4 x 10-7 ppb (parts per billion). This concentration is several orders of magnitude below the safe level for the most toxic chemicals.
From a physical perspective, the number of electric charges that would be deposited on the respiratory tract is also negligible in comparison with the concentration of electric charges naturally present in the mucus covering the bronchial cells or the concentration of electric charges located at the cell membrane.
Nevertheless, since the discovery of electrical charges in the air more than 100 years ago, there has been speculation suggesting that exposure to positive air ions in the natural environment may be adverse to one’s health while exposure to negative air ions may be associated with beneficial health effects. Most studies have focused on the respiratory system and mood and behavior. A few epidemiological studies have tested the hypothesis that air ions emitted by AC transmission lines would increase the deposition of pollutants in the lungs and on the skin of the residents living in the vicinity of the line, and increase the risk of cancer including leukemia. Results were inconclusive [22]. A comprehensive review of experimental data has concluded that neither positive nor negative ion exposure appears to have any clear physiological effects or potential for toxicity [14].
In summary, electric field levels and ion currents measured under existing lines up to 600 kV/m suggest that the probability for a person to experience some type of subtle sensory perception is low. However, perception is more likely to occur under hybrid AC and DC lines. These effects are not detrimental and do not constitute an adverse health effect.
3.6. Standards for human exposure to static electric fields
ICNIRP recommends no specific exposure limits to static electric fields or air ions. IEEE recommends an exposure limit for persons in unrestricted environments of 5 kV/m between 0 Hz and 368 Hz [8]. However, the rationale is to protect against electrostimulation effects, which do not occur for exposure to static electric fields.
3.7. Implanted cardiac pacemakers and defibrillators
There are no prescribed limits for the static electric field. The authors are not aware of any observations that would cast doubt on the immunity of these devices in the natural earth's electric field, which can reach several tens of kV/m in stormy weather. As a static electric field does not induce currents or voltages into the body, there are no plausible mechanisms to cause an interference.
4. Conclusion
In summary, the static magnetic field produced by an HVDC line is of the same order of magnitude as the natural magnetic field. The levels of the Earth’s magnetic field will be distorted by the presence of the DC line but this distortion (a decrease or an increase) will be generally limited to a distance of a few tens of meters from the centerline. The resulting magnetic field is of the same order of magnitude as the earth magnetic field which is about 2 000 times weaker than the most restrictive exposure limit for the public (50 µT vs. 118,000 µT). There is no evidence that the ubiquitous natural magnetic field causes significant physiological or pathological effects in humans. No impact on human health is anticipated.
In the particular case of a single underground HVDC cable buried with a reduced depth, magnetic field levels at ground level may approach the recommended limit to avoid interference with cardiac implants. Attention to this particular situation should be paid when designing an underground HVDC line.
In the absence of significant corona discharges, the electric field produced by HVDC lines will remain imperceptible at almost all times even to the most sensitive individuals. In the presence of corona discharges, the perception threshold may be lower depending on the current density. Under an HVDC line producing a current density of 50 to 100 nA/m² or more, the electric field may be perceptible through discrete hair movements at the surface of the skin.
It is unlikely that a person under the line will experience a micro-discharge on contact with a conducting object. If this phenomenon were to occur, it may be surprising, but it is harmless. There are no known harmful effects from static electric fields.
In conclusion, the static electric and magnetic fields produced by the HVDC line are safe and there is no scientific evidence that they pose a risk to human health.
References
- M. Plante, J. Lambrozo, M. Souques, D. C. Brown, P. Arnera, J. A. Bulcao, and S. Nakasono, “50-60 Hz magnetic fields and cancer, forty years of research: it is time to reassure,” Working Group Report Ref. WGR_287_2, CIGRE WG C3.01, ELECTRA, 2016.
- Measurement of DC magnetic, AC magnetic and AC electric fields from 1 Hz to 100 kHz with regard to exposure of human beings – Part 2: Basic standard for measurements, IEC Standard IEC 61786-2, Dec. 2014.
- B. Pophof, B. Henschenmacher, D. R. Kattnig, J. Kuhne, A. Vian, and G. Ziegelberger, “Biological Effects of Electric, Magnetic, and Electromagnetic Fields from 0 to 100 MHz on Fauna and Flora: Workshop Report,” Health Phys, vol. 124, pp. 39-52, 2023.
- International Commission on Non-Ionizing Radiation Protection, “Guidelines on limits of exposure to static magnetic fields,” Health Phys, vol. 96, pp. 504-514, 2009.
- J. F. Schenck, “Physical interactions of static magnetic fields with living tissues,” Prog Biophys Mol Biol, vol. 87, pp. 185-204, 2005.
- Council of the European Union, “Council recommendation of 12 July 1999 on the limitation of exposure of the general public to electromagnetic fields (0 Hz to 300 GHz),” Official Journal of the European Communities, vol. 42, pp. 59-70, 1999.
- International Commission on Non-Ionizing Radiation Protection, “Guidelines on limits of exposure to static magnetic fields.,” Health Phys, vol. 66, pp. 100-106, 1994.
- IEEE Standard for Safety Levels with Respect to Human Exposure to Electric, Magnetic, and Electromagnetic Fields, 0 Hz to 300 GHz, IEEE Standard C95.1-2019, 2019.
- European Parliament and Council of the European Union, “Directive 2013/35/EU of the European Parliament and of the Council of 26 June 2013 on the minimum health and safety requirements regarding the exposure of workers to the risks arising from physical agents (electromagnetic fields) (20th individual Directive within the meaning of Article 16(1) of Directive 89/391/EEC) and repealing Directive 2004/40/EC,” Official Journal of the European Union, vol. 56, pp. 1-21, 2013.
- Procedure for the assessment of the exposure to electromagnetic fields of workers bearing active implantable medical devices – Part 2-2: Specific assessment for workers with cardioverter defibrillators (ICDs), CENELEC Standard EN 50527-2-2, May 2018.
- A. Bennett, "Measurement of Atmospheric Electricity During Different Meteorological Conditions," Doctor of Philosophy, Department of Meteorology, University of Reading, 2007.
- A. Yu, S.-L. Lai, Y. Chen, and M. Shi, “Application of ground electric field in smart power supply against lightning of wireless access base station,” in Proc 2005 International Symposium on Electromagnetic Compatibility, Chicago, pp. 364-367 Vol. 2.
- T. D. Bracken and G. B. Johnson, "Small Air Ion Environments," in Air Ions - Physical and Biological Aspects J. M. Charry and R. Kavet, Eds., ed Boca Raton: CRC Press, 1987.
- W. H. Bailey, A. L. Williams, and M. J. Leonhard, “Exposure of laboratory animals to small air ions: a systematic review of biological and behavioral studies,” Biomed Eng Online, vol. 17, p. 72, June 2018. Available: 10.1186/s12938-018-0499-z.
- R. D. Dallaire and P. Jutras, “Suivi environnemental ligne C.C. Des Cantons/Comerford 450 kV (Ascot),” Hydro-Québec, Montréal, Rapport interne IREQ #4545 1989.
- P. S. Maruvada, “Electric Field and Ion Current Environment of HVdc Transmission Lines: Comparison of Calculations and Measurements,” IEEE Transactions on Power Delivery, vol. 27, pp. 401-410, 2012.
- P. S. Maruvada, R. D. Dallaire, P. Heroux, and N. Rivest, “Long-Term Statistical Study of the Corona Electric Field and Ion-Current Performance of a ±900-kV Bipolar HVDC Transmission Line Configuration,” IEEE Transactions on Power Apparatus and Systems, vol. PAS-103, pp. 76-83, 1984.
- CIGRE WG C4.25, “Issues related to spark discharges,” 475, 2018.
- A. K. Petri, K. Schmiedchen, D. Stunder, D. Dechent, T. Kraus, W. H. Bailey, and S. Driessen, “Biological effects of exposure to static electric fields in humans and vertebrates: a systematic review,” Environ Health, vol. 16, p. 41, 2017.
- J. P. Blondin, D. H. Nguyen, J. Sbeghen, D. Goulet, C. Cardinal, P. S. Maruvada, M. Plante, and W. H. Bailey, “Human perception of electric fields and ion currents associated with high-voltage DC transmission lines,” Bioelectromagnetics, vol. 17, pp. 230-241, 1996.
- M. Kursawe, D. Stunder, T. Krampert, A. Kaifie, S. Drießen, T. Kraus, and K. Jankowiak, “Human detection thresholds of DC, AC, and hybrid electric fields: a double-blind study,” Environ Health, vol. 20, p. 92, 2021.
- Agence nationale de sécurité sanitaire de l’alimentation de l’environnement et du travail (ANSES), Avis de l’Anses - Rapport d’expertise collective : Effets sanitaires liés à l’exposition aux champs électromagnétiques basses fréquences: ANSES editions, 2019.
