C1 - The challenges of developing electrolysis for the French power system in the medium to long term
Authors
Marc LE DU, Mathilde FRANCON, Marie-Alix DUPRE LA TOUR, Cédric LEONARD, Baptiste MENARD - RTE, France

Summary
The role of hydrogen has become increasingly important in discussions on the energy transition. RTE has analyzed the challenges of developing electrolysis in France in several studies carried out in recent years.
By 2030-2035, hydrogen should be contributing to the decarbonization of the economy. Hydrogen production by electrolysis, in line with the national strategy, represents a decarbonized electricity requirement of around 30 TWh in 2030 to 50 TWh in 2035, for industrial uses, mobility and the manufacture of synthetic fuels. The electricity system projected for 2035 is capable of accommodating these new uses: growth in renewable production should outstrip the increase in consumption, including the production of hydrogen by electrolysis. From the point of view of power demand and security of supply, electrolysis units are considered flexible, and will be able to stand down when the electrical system is under stress, provided that hydrogen-powered industrial processes are able to adapt to such interruptions. Several operating modes can be envisaged, ranging from base operation to self-production using renewable energy. Whatever the mode, the price of decarbonated hydrogen produced by electrolysis appears to be higher than that of steam reforming of natural gas. The sector's development will therefore depend on changes in taxation and public support.
The use of Power-to-Gas-to-Power is an option to be considered beyond 2035, for mixes with a very high proportion of renewable energy. For scenarios with up to 100% renewable energy, the quantities needed for this balancing would reach up to 35 TWh of hydrogen, requiring adaptation of the production facilities for domestic production of this hydrogen. The impact of electrolysis on electricity prices in decarbonized mixes is uncertain. Analyses by RTE suggest that prices could often be shaped by demand for electrolysis, through arbitrage with extra-European imports of hydrogen, in contrast to the common view of mostly zero prices. The green gases needed for balancing will also lead to periods of very high electricity prices.
Keywords
Hydrogen, Flexibility, wind, solar, nuclear, demand flexibility, power-to-gas, gas networks and storage, electricity prices1. Hydrogen: a challenge for the energy transition
In recent years, the role of hydrogen has become increasingly important in discussions on the energy transition. In France, at the end of 2023, the government published for consultation its new strategic guidelines for the development of decarbonized hydrogen [1].
This orientation reflects the ambition to structure a real hydrogen industry, supporting the development of an important building block for the energy transition. Low-carbon hydrogen offers a solution for reducing emissions in the industrial and transport sectors, as well as for the use of natural gas in the grid. In the longer term, the production and storage of low-carbon hydrogen could offer a complementary solution for the controllable production of electricity to meet the system's flexibility needs, particularly interesting in scenarios with a high proportion of renewable energies.
RTE has analyzed these challenges for the development of electrolysis in France in several studies carried out in recent years, based on large-scale models of the European power system over different time horizons.
An initial study published in 2020 was part of a work program on new uses for electricity: electric mobility [2] heating in the building sector [3] and hydrogen production by electrolysis by 2035 [4]. The outlook for electrolysis in this timeframe was reviewed and clarified in the recent Bilan prévisionnel published at the end of 2023 [5].
The prospects for carbon neutrality were analyzed in the Energy Pathways 2050 study published in 2022. [6], [7]. Beyond the decarbonization of certain uses, the aim was to analyze the challenges of developing Power-to-Gas-to-Power to contribute to the flexibility needs of an electricity system based massively on non-controllable renewable energies. Such developments in electrolysis, and the reuse of hydrogen for the power system, lead to a highly coupled operation of two energy systems. Coordinating the production, storage and other flexibilities of each of the two vectors is therefore fundamental. It raises questions about the overall optimization of the energy system and the coordinated formation of prices on its various energy vectors, analyzed as part of a thesis to be defended in 2023. [8].
This article presents a summary of the main results of these analyses and their outlook.
2. Challenges for 2035: contributing to the decarbonization of the economy
2.1. Decarbonized hydrogen: an attractive alternative to fossil fuels in sectors that are difficult to decarbonize
At present, the hydrogen consumed in France corresponds almost exclusively to non-energy industrial uses, mainly in the oil refining, ammonia production and chemical sectors. The hydrogen used in these processes is essentially produced using fossil fuels (95% from gas, oil and coal), which emit CO2 . Every year, France produces around 400,000 tonnes of hydrogen from dedicated sources, in particular the steam reforming of methane, leading to the emission of around 5 million tonnes of CO2. One of the priorities identified by the French government for the development of hydrogen is therefore to convert conventional industrial hydrogen production to a low-carbon production method.
Another medium-term objective for hydrogen is to facilitate the decarbonization of sectors that are difficult to electrify directly, such as heavy mobility, air and sea transport, and the steel industry. In these different sectors, hydrogen can enable the avoidance of fossil fuels, through the use of fuel cells for heavy mobility, the development of alternative fuels for aviation (synthetic liquid fuels) and shipping (ammonia), or the use of direct iron ore reduction techniques for the steel industry.
In any case, the first step is to develop significant volumes of low-carbon hydrogen production in France over the next decade. This development will be based largely on water electrolysis, powered by electricity, which has the advantage of already being largely carbon-free in France (around 95%). The government's objective is to produce around 600,000 tonnes of carbon-free hydrogen per year by 2030, and one million tonnes by 2035.
2.2. The power system projected to 2035 is capable of accommodating the development of electrolysis
Electrolysis is not the only solution for producing carbon-free hydrogen. Other solutions can be envisaged, such as steam reforming of biomethane, or steam reforming of natural gas with carbon capture and storage. However, these solutions raise issues of biomass resource availability for the former, and logistics, long-term carbon storage capacity and acceptability for the latter. France therefore favors the use of water electrolysis, facilitated by an electricity mix that is already highly decarbonized.
Hydrogen production by electrolysis, corresponding to the national strategy, represents a decarbonized electricity requirement of around 30 TWh in 2030 and 50 TWh in 2035. The government's objective is to deploy 6.5 GW of low-carbon electrolytic hydrogen production capacity by 2030 and 10 GW by 2035. This increase in electricity consumption is compatible with France's ambitions to develop its carbon-free power generation fleet.
As shown in Figure 1decarbonated electricity production (nuclear and renewable) was higher than domestic consumption (including losses) in 2019. In the following years, nuclear production fell due to the shutdown of the Fessenheim generating units, the disorganization of maintenance programs due to the Covid crisis, and then the lower availability of the nuclear fleet due to stress corrosion problems. However, consumption has also fallen as a result of the economic crises and the sobriety measures taken since 2022 in response to energy prices. The improvement in production in 2023 enabled this consumption to be covered by decarbonized electricity.
The French government's objectives of decarbonizing and reindustrializing the French economy call for strong growth in electricity consumption, of which electrolysis is a major part. Other increases in consumption are due to the rapid electrification of the fleet of electric vehicles, as well as a proportion of heavy goods vehicles, the numerous projects to decarbonize existing industrial sites, the establishment of new factories (particularly for batteries), the development of data centers, etc. For heating, despite a sharp acceleration in the deployment of alternatives to oil- and fossil-gas-fired boilers, the increase in consumption would be limited, given the high efficiency of electric heat pumps and the savings made at the same time by renovation. These prospects are not specific to France, but are reflected in all decarbonization strategies in Europe, as part of the European REPowerEU plan.
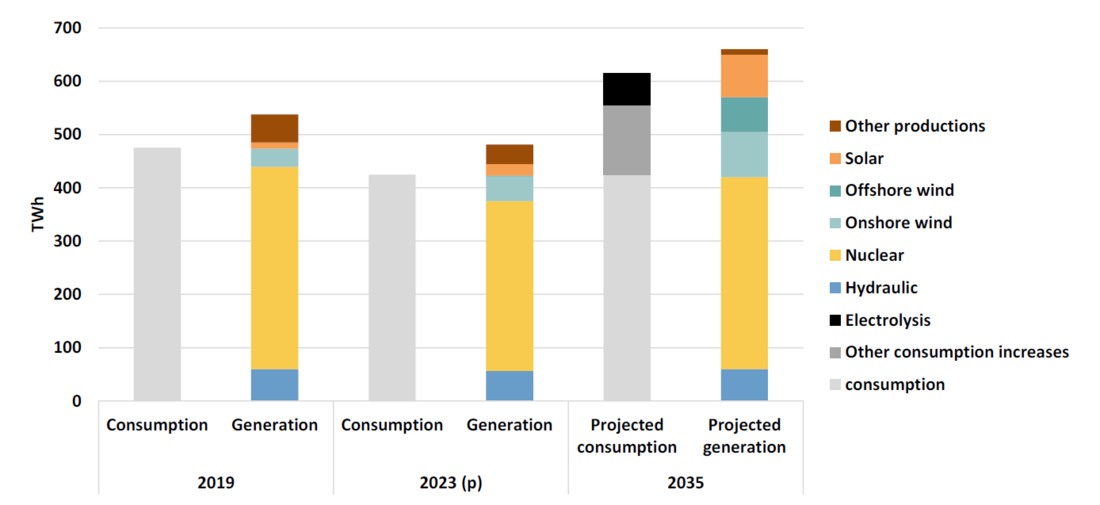
Figure 1 - Evolution of electricity consumption and generation to 2035 in the reference scenario
This increase in consumption must be accompanied by new, low-carbon generation. The French government has decided to gradually renew its nuclear generation fleet, but the commissioning of new units before 2035 will be limited to the EPR reactor at Flamanville. The increase in decarbonized production will be based almost exclusively on renewable energies: between now and 2030, it will mainly be onshore wind and solar production that will be commissioned; offshore wind farms, the first of which was commissioned in 2022, will be deployed mainly after 2030. The growth in these renewable energy sources should outstrip the increase in specific consumption, including the production of hydrogen by electrolysis.
From the point of view of power demand and security of supply, electrolyzers are considered flexible and will be able to stand down in the event of a power outage, provided that hydrogen-powered industrial processes are able to adapt to these interruptions. [9] [10] [11] [12].
2.3. Gains on CO2 emissions are clear from a national accounting perspective
Electricity generated in France is already largely carbon-free. This configuration is conducive to the development of new uses such as electrolysis as a substitute for processes that emit more greenhouse gases.
In the reference scenario presented, the substitution of fossil hydrogen or fossil fuels by hydrogen produced by electrolysis or derived fuels will reduce direct CO2 emissions from industry and transport in France (scope 1) by around 8 MtCO2/year by 2030 and 18 MtCO2/year by 2035. The contribution of the development of electrolysis to the reduction of national CO2 emissions comes from the avoidance of the use of natural gas for steam reforming, the avoidance of coal combustion in the steel industry and the substitution of petroleum products by decarbonated hydrogen or derived synthetic fuels in transport. They do not take into account indirect emissions from the production of fossil fuels, linked to their extraction, transport, distribution, possible leaks, etc.
But assessing the environmental benefits of using hydrogen produced by electrolysis cannot be limited to an analysis of the direct CO2 emissions avoided. It is also necessary to account for the emissions that the development of electrolysis induces on electricity production (scope 2). Analysis of the effect on emissions must necessarily take into account the interconnection of the French system with its neighbors, as well as the evolution of the mix concomitant with the development of new uses for electricity.
With an unchanged generation mix, the analysis on a European scale would be more nuanced. All other things being equal, the extra electricity consumption in France (to power electrolysers) would lead to a reduction in exports of low-carbon electricity to other countries. Yet exporting decarbonated electricity to avoid coal- or gas-fired power plants saves more CO2 than substituting electrolysis for natural gas steam reforming to produce hydrogen, as illustrated in Figure 2. If electrolysis were to take place at the expense of electricity exports, avoiding the operation of fossil-fired generating units, the balance sheet in terms of CO2 emissions on a European scale would therefore have to be put into sharp perspective.
However, this effect is counterbalanced by the increase in decarbonized electricity production planned by the public authorities. By integrating this adaptation, which increases decarbonized production as new uses for electricity such as electrolysis are developed, electricity exports are maintained. Even if these exports were to decrease, it would be legitimate to question the environmental trade-off between using electricity in France to decarbonize its own economy and exporting electricity to decarbonize the electricity mixes of neighboring countries. The international framework for assessing greenhouse gas emission reduction trajectories is based on territorial accounting. From this point of view, the gains in CO2 emissions associated with the development of electrolysis in France are very clear.
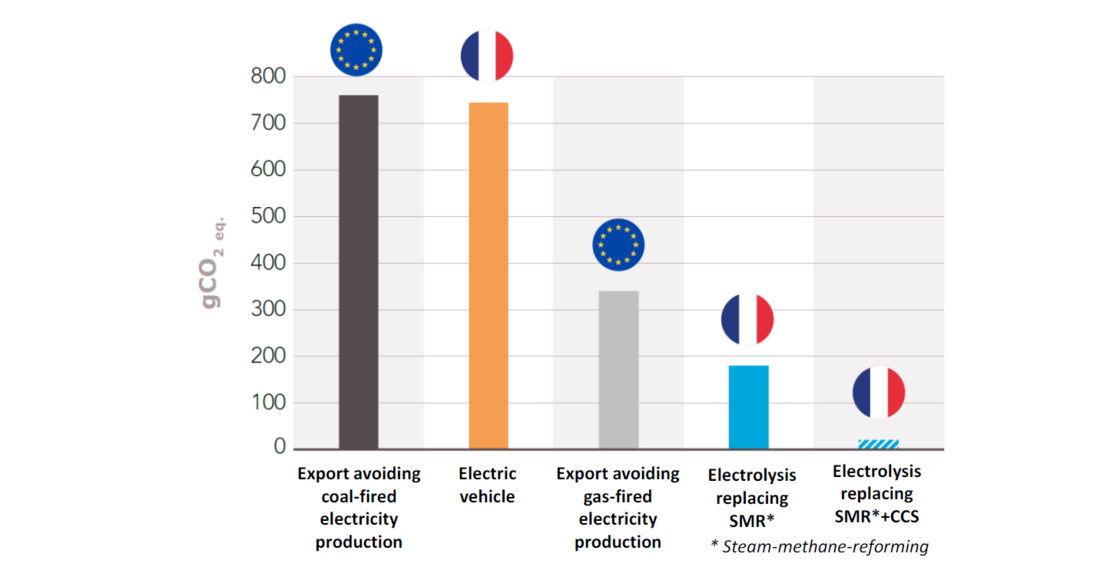
Figure 2 - Emissions avoided by the production of 1 kWh of decarbonized electricity in France, depending on whether it is used in France or in Europe
2.4. For the players involved, the economic interest systematically depends on public support, and incorporates many parameters other than the cost of the electrolyzers.
An analysis of the player models envisaged for decarbonated hydrogen production in France suggests several possible operating modes for electrolyzers. Three operating modes, deliberately very distinct, are explored in RTE's studies and illustrated in Figure 3 :
- market supply during periods of renewable or nuclear "surplus";
- supply on the base electricity market, except if interruption is needed;
- coupling with renewable generation (e.g. photovoltaic) as part of "local" models.
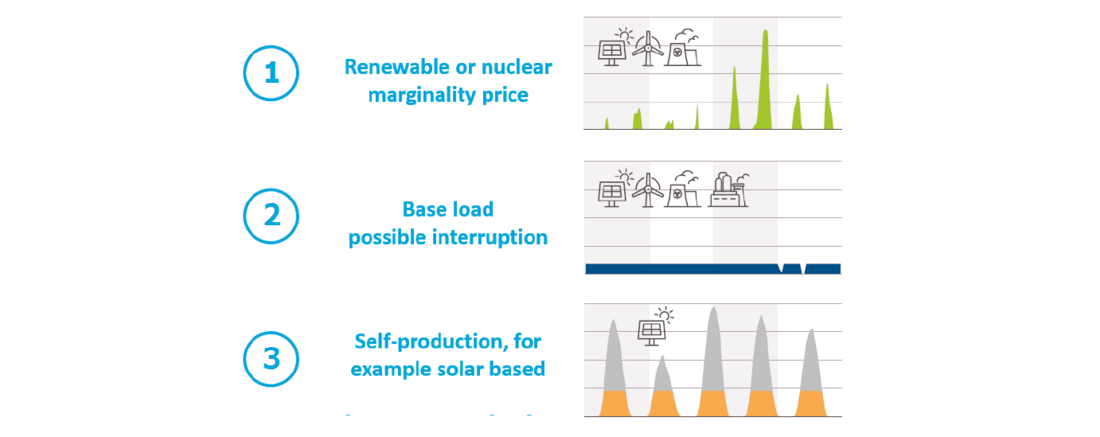
Figure 3 - Three electrolysis operating modes used in the analyses
These operating modes lead to very different electrolyzer load factors and technical and economic challenges.
To produce competitive hydrogen, electrolyser operators are likely to seek a compromise over the operating period between amortization of fixed costs (favoring a long annual operating period) and access to low electricity prices (favoring operation centered on the hours of lowest prices). For industrial applications, however, deviating from base load supply can lead to additional costs, depending on the intended use. If electrolyser operation is not permanent, a storage solution (or hydrogen process flexibility) must be provided. This needs to be factored into the economic analysis and costs of switching to low-carbon hydrogen.
Operating methods are characterized by different sensitivities to economic parameters. The figures shown in Figure 4 correspond to European economic conditions and production mixes in 2035, projected to 2020. These conditions have changed since this study, due to inflation, tensions in the supply chains of certain components, and the upward revision of the rate of inclusion of renewable energies in the European generation mix called for by the RepowerEU strategy. While the numerical values may have changed, the orders of magnitude and cost sensitivities to the main parameters remain valid.
- For mode 1 (market supply in situations of surplus renewable or nuclear energy), the price of electricity on the wholesale market has little effect on the economic equation, as operation is by design centered on periods of low price. Nevertheless, this mode of production implies reduced operating times (load factor of 10 to 15%), leading to increased sizing of electrolyzers for the same hydrogen production, and possibly the development of a downstream hydrogen distribution chain incorporating dedicated storage facilities needed to compensate for the variability of electrolyzer operation. The production cost of this operating mode is therefore highly sensitive to the cost of electrolyzers and hydrogen storage. This mode of operation does not appear to be competitive with the other two by 2035.
- For mode 2 (base supply on the electricity market), the cost of electrolysers does not appear to be a determining factor, which may put the current debate on the evolution of investment costs for electrolysis facilities into perspective. Rather, the issue identified by the study concerns access to low electricity prices. Paradoxically, the increase in the price of carbon on the European ETS market is not conducive to the supply of electrolysis on electricity markets: indeed, as long as marginal power plants on the European electricity system run on gas or coal, this price will be high, not reflecting the costs of producing low-carbon electricity. Thus, the increase in this price ultimately leads to a rise in the cost of electricity supply, irrespective of the French production mix, and thus penalizes the production of low-carbon hydrogen by electrolysis. Stakeholders are therefore looking for a way to insensitize supply costs to CO2 and fossil fuel prices, via PPAs for example. This is the operating method currently favored in France.
- For mode 3 (coupling with dedicated production), the main determinant of the economic model is the full cost of the renewable production facilities coupled to the electrolyzers. The operating mode simulated in this study is mixed, with the hydrogen producer selling on electricity markets the renewable production not used for electrolysis. This model therefore generates revenues which, unlike mode n°2, interest the hydrogen producer at high electricity prices. However, the uncertainty surrounding European market prices, particularly during periods of high photovoltaic production, makes this outlet uncertain. More economical renewable production conditions in other parts of the world could make this model more attractive from a hydrogen import perspective, although transport costs would have to be factored into the trade-off.
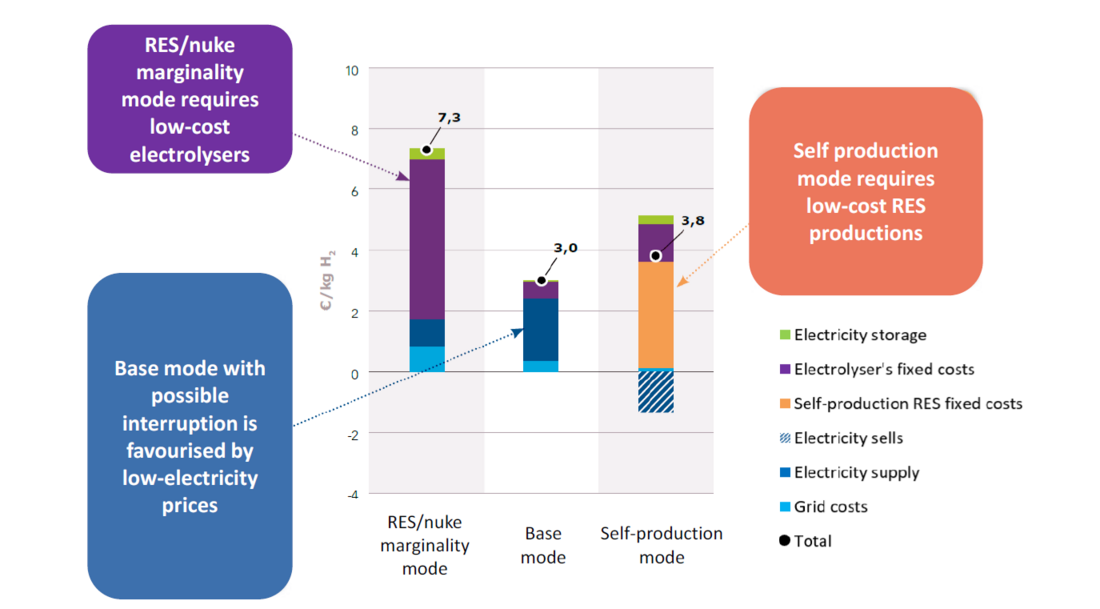
Figure 4 - Cost of production in 2035 for the hydrogen producer according to electrolyzer operating mode (economic conditions as seen in 2020, in €2020)
With an unchanged regulatory and pricing framework, and unless the price of CO2 reaches extremely high levels, the price of decarbonated hydrogen produced by electrolysis would appear, in all three modes, to be higher than that of natural gas steam reforming, even allowing for significant reductions in electrolyser costs. The sector's development will therefore depend on changes in taxation and public support.
3. Hydrogen storage to ensure power system balance, a longer-term challenge
3.1. Power-to-gas-to-power does not seem necessary before 2035
Hydrogen is also presented as a storage and retrieval solution that is necessary to balance the electricity system, following the Power-to-gas-to-Power principle. Hydrogen plays the role of a "buffer": it is produced by electrolysis from decarbonized electricity, it is stored (e.g. in salt caverns or in gas systems after conversion into synthetic methane), and it is (re)transformed into electricity during periods of low wind or solar production. It can then become an inherent component of the power system, like today's controllable generation resources.
However, by 2035, hydrogen storage is not essential to meet the need to compensate for the variability of renewable energies. RTE's analyses of different scenarios for this timeframe show that the introduction of a greater proportion of non-controllable wind and photovoltaic electricity will increase the need for flexibility by 2035. However, the European power system will still have controllable generation units: nuclear, hydro, fossil-fired or renewable (biomethane, etc.). To compensate for the reduced flexibilities provided by fossil-fired generation, the development of consumption flexibilities is favored: new uses for electricity should lend themselves to this: electric vehicles, domestic hot water, etc. and electrolysis. We also expect to see the development of stationary batteries, in particular to support the growth of photovoltaic production.
The low energy efficiency of power-to-gas-to-power (between 25% and 35% depending on current technologies) and its high costs make it uncompetitive with these solutions in the medium term. At these times, the use of hydrogen as a means of "electricity storage" is therefore unnecessary.
3.2. An option to consider in view of the high proportion of renewable energies in the production mix
On the other hand, its study is of great interest in the longer term (to 2050). Scenarios based exclusively or predominantly on renewable energies will necessarily need to rely on balancing resources, including various forms of storage. In the Energy Pathways 2050 study [6] [7] RTE analyzed the flexibilities required for different electricity generation mixes in France, ranging from 50% renewable energy (and 50% nuclear) to 100% renewable energy, for a final electricity consumption of 650 TWh.
For this analysis, simulations of hourly balances in the European power system have been extended to other energy carriers, in particular hydrogen. These simulations are carried out using the ANTARES simulator developed by RTE [13].
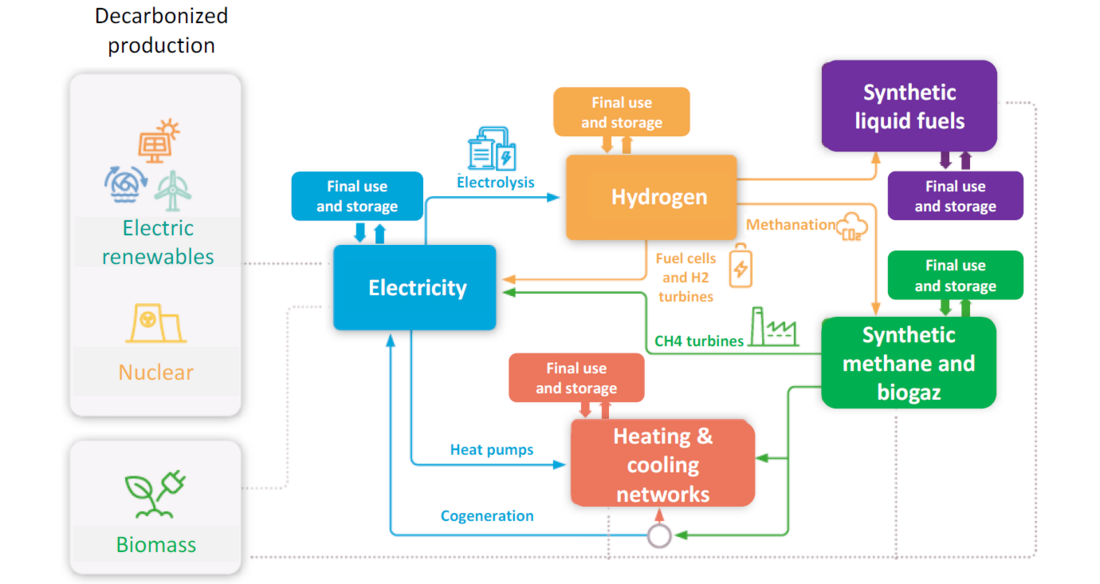
Figure 5 - Main interactions between electricity and other vectors in an energy system based on decarbonized electricity and biomass
These analyses show that the need for decarbonated gas to balance the system, in addition to other means (batteries, demand-side management, load shedding, hydropower, nuclear power, flexibilities provided by interconnections) depends very much on the type of renewable generation and the proportion of nuclear power in the fleet. The Figure 6 illustrates the corresponding hydrogen requirements, assuming that these decarbonized gases are solely syngas (pure hydrogen or synthetic methane). Assuming a power-to-methane-to-power loop, the quantities of hydrogen required are greater, due to the additional loss of efficiency in methanation. The use of pure hydrogen for electricity generation presupposes that turbines adapted to this fuel will be installed in GCC and TAC equipment in the near future.
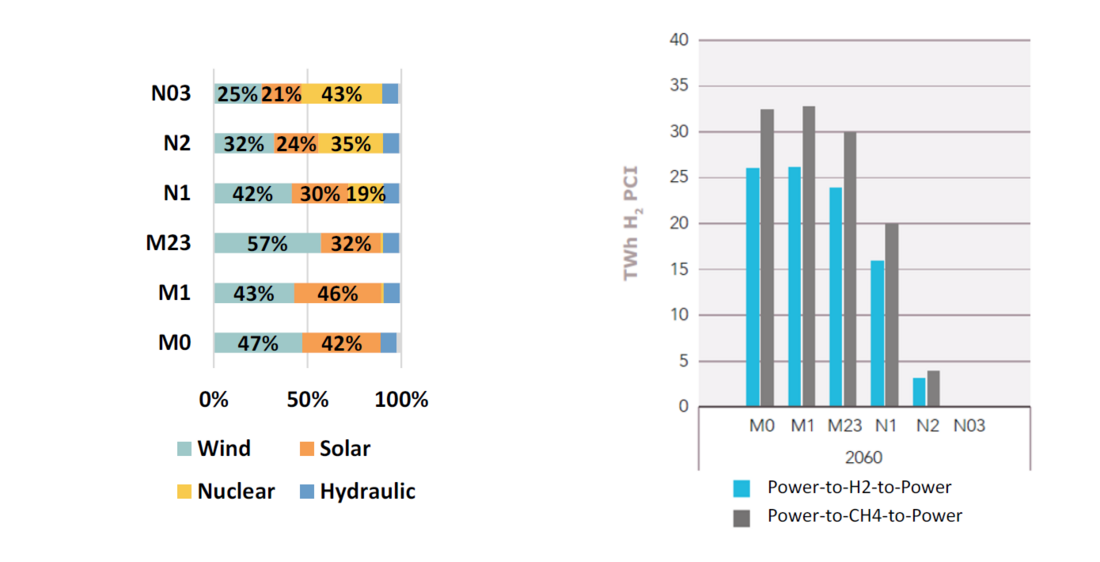
Figure 6 - Shares of types of production in different scenarios of French electricity mix in 2060 (left)
and associated requirements on hydrogen needed for seasonal balancing of the carbon-free electricity system (right)
On the carbon-neutral horizon, the need for decarbonated gas to balance the power system is driven by seasonal requirements. This explains why the greater the share of nuclear power in the production mix, the lower these requirements will be. Because of heating needs, energy consumption in France and most other European countries is higher in winter than in summer (unlike in many other parts of the world, where the greatest need is for air conditioning in summer). Wind generation, which is more important in winter, is therefore better suited to this seasonal pattern than photovoltaic generation: the greater the share of photovoltaic in the generation mix, the greater the need for low-carbon gases.
3.3. Adaptation of the generating fleet to take account of these balancing requirements, which affect hydrogen costs
RTE's analyses assume that all the decarbonated gases needed for balancing are produced from hydrogen, and that this hydrogen is produced by electrolysis in France. Over and above the production planned to satisfy final electricity consumption, the fleet must therefore be adapted to supply this additional consumption, taking into account hydrogen and electricity production efficiencies. For 1 TWh of electricity returned to the power system, an overall efficiency of 35% implies additional electricity production of around 2.8 TWh.
In the 100% renewable scenario, with 57% wind power and 32% photovoltaics, 34 TWh of additional renewable generation must be provided to balance the system. As this additional generation also modifies the system's flexibility requirements, an iterative calibration of the generation and balancing mix must be carried out.
The Figure 7 illustrates the cost of hydrogen needed to balance the electricity system, for economic conditions in 2020.
This is not the cost price as seen by one player, as analyzed in § 2.4but the sum of collective costs in relation to the quantities of hydrogen produced. These collective costs include a share of electricity system costs (production and networks) proportional to the quantities of electricity produced, electrolysis costs and costs incurred in storing and transporting hydrogen. This last item assumes that hydrogen infrastructure costs would be around 3 times higher than natural gas infrastructure costs, corresponding to the energy density ratio of hydrogen to methane.
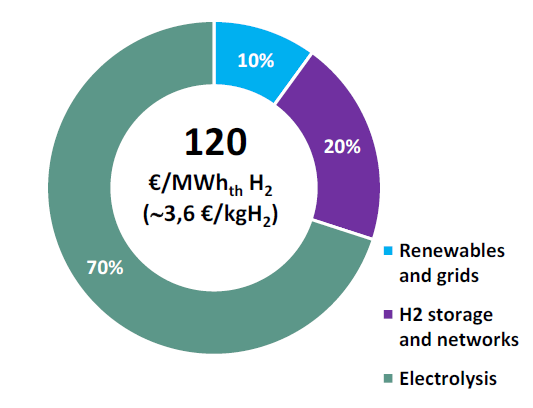
Figure 7 - Estimated costs of hydrogen production, transport and storage on the carbon neutrality horizon (€2020)
This evaluation obviously depends on an assessment of the respective costs of renewable production, electrolysers and hydrogen infrastructure. However, this level of €120/MWhH2-PCI seems a reasonable order of magnitude for the collective cost of European hydrogen. Lower production costs could be envisaged in non-European countries, but transport costs would then have to rise.
3.4. The impact of energy coupling on electricity prices
Assuming that this order-of-magnitude cost of hydrogen is reflected in the selling price of decarbonated gases for electricity production, the order-of-magnitude variable cost of production would be around €240/MWhelec , assuming a production unit efficiency of around 50%.
It therefore seems unfounded to think that high electricity prices, driven by marginal production costs, are solely the result of the use of fossil gases. Decarbonated gases will logically be expensive, as their production, transport and storage require substantial investments, even greater than those needed to extract fossil gases. In view of the uncertainties that climate change poses for the world's biomass resources, which are also highly coveted for many uses, the alternative of producing electricity from biogas cannot guarantee lower costs.
In addition, work carried out at RTE as part of a doctoral thesis [8] suggests that, in a coupled energy system, decarbonized gas prices would influence almost the entire electricity market price curve. This is obvious during periods of decarbonated gas power generation, as in the case of fossil gas use today. But the generalization of hydrogen production by electrolysis, whether for end uses or for "electricity storage", induces an economic trade-off with possible imports of decarbonated gases.
Indeed, even when the marginal cost of electricity production is zero (100% renewable production, for example), the demand for electrolysis has no reason to be supplied free of charge. For hydrogen consumers, the alternative to domestic production by electrolysis would be to import hydrogen. They would therefore have to be prepared to buy local hydrogen for the same price, i.e. pay for the electricity supplied for electrolysis at the price of imported hydrogen multiplied by the electrolysis yield. If an electricity supplier were to offer a lower selling price to a hydrogen producer, this would be an obvious economic arbitrage. In turn, this would also lead to a price for electricity demand that would also apply to other controllable consumption, such as electric vehicle charging.
Under these conditions, the price of electricity corresponds to the marginal cost of demand, rather than the marginal cost of supply as under current conditions. This is shown by the coupled energy system simulations carried out by RTE, which calculate the marginal costs of the various energy vectors. Another way of explaining this price formation mechanism is to consider the consequences of an increase in electricity consumption of 1 MWh (this is the definition of marginal cost). If this increase occurs during a period of hydrogen production by electrolysis, this production must be reduced by 0.7 MWhH2 (assuming an efficiency of 70%), and the corresponding volume must be imported. The marginal cost to the energy system of increased electricity consumption is therefore equal to the cost of importing hydrogen multiplied by the efficiency of electrolysis.
The Figure 8 illustrates the shape of the price monotone obtained, showing three distinct zones:
- The high-price zone (purple) corresponds to electricity generation from decarbonized gases, based on the prices of the various gases and the efficiency of electricity generation facilities.
- The central (green) zone corresponds to periods of flexible electricity demand, when the electrolyzers can operate at the margin of renewable or nuclear generation.
- The low-price zone (yellow) corresponds to periods of surplus renewable or nuclear generation.
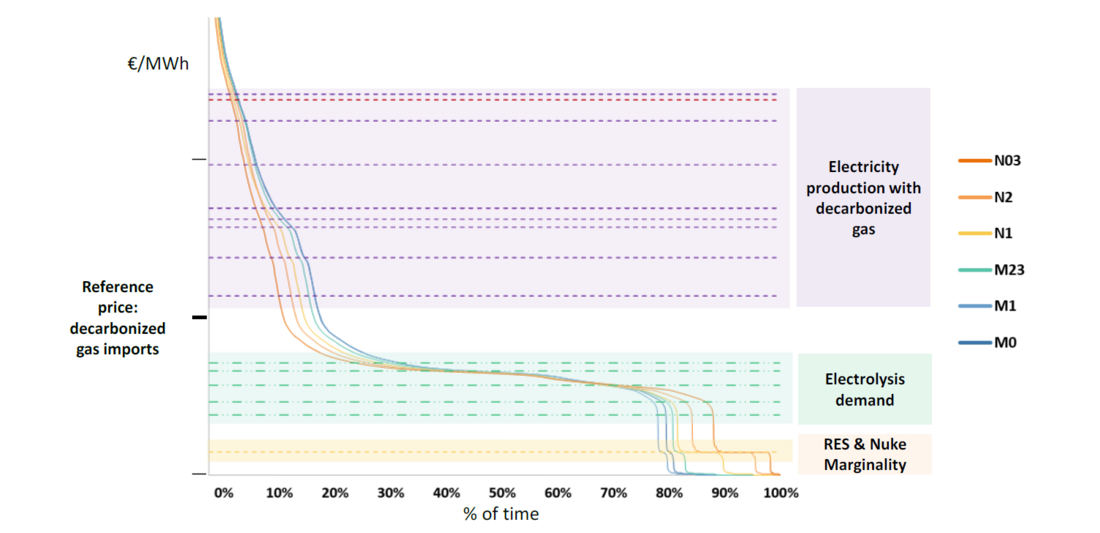
Figure 8 - Electricity price monotone in an energy system coupled with decarbonized power generation
The absence of clear steps on this price curve is due to the smoothing effect of various forms of storage (hydro, batteries) and, as mentioned above, other demand flexibilities than electrolysis.
This effect of flexible electricity demand on market prices needs to be confirmed. In particular, it does not include any representation of the behavior of market players in relation to electricity exchanges, but is based solely on an analysis of the marginal costs of the optimized system. This would represent a clean break with the common position, which considers that market prices for such generation mixes would be zero for a very large part of the time (for example [14]).
4. Conclusion
This paper presents some of the findings of analyses carried out by RTE on the challenges associated with hydrogen production by electrolysis for the French power system. They clarify certain aspects of the envisaged uses, environmental impacts, operating methods and economics associated with the development of electrolysis. Over the longer term, they examine the role that hydrogen could play in balancing the electricity system and determining electricity prices.
However, many points remain wide open.
With regard to hydrogen infrastructures, the French strategy calls for all major industrial zones to have hydrogen production capacity by 2030. Priority is given to the development of networks within hubs corresponding to the major industrial zones of sea and river ports, and their connection to storage infrastructures. However, local deployment is still necessary, particularly to supply land-based mobility applications. This implies the creation of a territorial network for the refueling of alternative fuels, in line with the European AFIR regulation [xx], based as far as possible on industrial hubs. In the longer term, these local networks of industrial hubs could be interconnected as part of the development of a European hydrogen network. [15] and in particular as part of the H2Med project to link Portugal, Spain, France and Germany.
These infrastructures make it possible to envisage different sources of hydrogen supply. Water electrolysis, currently the preferred source, is not the only possible solution. In particular, France is launching an exploratory study into natural hydrogen, to assess its extraction potential, economic interest and environmental impact. The potential of the French territory will be evaluated for 2025. The possibility of non-European imports may also be considered, subject to their economics, environmental impact and transport implications. Last but not least, the possibility of locating electrolysers close to major renewable production sites, such as off-shore wind farms, also depends on the development of these infrastructures.
The prospects for using decarbonated gases to support the electricity system are still a long way off, but they do raise the question of infrastructure development. Alternatives to hydrogen exist, such as biogas and synthetic methane. Given the uncertainties surrounding biomass deposits as a result of climate change, and the covetousness they generate for many uses, the use of biomass for electricity generation is likely to remain marginal. Synthetic methane has also been mentioned as an alternative. This technology could be based on existing natural gas network and storage infrastructures, but requires the development of a carbon supply infrastructure and logistics, necessary for methanation.
These various issues will need to be investigated as part of these studies, in order to define the shape of the future coupled energy system.
References
- French Government, «Stratégie nationale pour le développement de l'hydrogène décarboné en France,» pour consulation, décembre 2033.
- RTE, «Enjeux du développement de l'électromobilité pour le système électrique,» RTE, Paris, 2019.
- RTE - ADEME, «Réduction des émissions de CO2, impact sur le système électrique : quelle contribution du chauffage dans les bâtiments à l'horizon 2035 ?,» RTE - ADEME, Paris, 2020.
- RTE, «La transition vers un hydrogène bas carbone : atouts et enjeux pour le système électrique à l'horizon 2035,» RTE, Paris, 2020.
- RTE, «Bilan prévisionnel 2023 : 2023-2035, première étape vers la neutralité carbone - Synthèse,» 2023.
- RTE, «Energy pathways 2050 - key results,» 2022.
- RTE, «Futurs énergétiques 2050 - rapport complet,» 2022.
- M.-A. D. l. Tour, «Towards a decarbonized energy system in Europe in 2050 : impact of vector coupling and renewable deployment limits,» PHD report, 2023.
- Digvijay Gusain; Miloš Cvetković; Ron Bentvelsen; Peter Palensky, «Technical Assessment of Large Scale PEM Electrolyzers as Flexibility Service Providers,» IEEE 29th International Symposium on Industrial Electronics (ISIE), n° %1doi: 10.1109/ISIE45063.2020.9152462, pp. pp. 1074-1078, 2020.
- Martin Roach, Leonardo Meeus, «The welfare and price effects of sector coupling with power-to-gas,» Energy Economics, n° %1doi:10.1016/j.eneco.2020.104708, 2020.
- Oliver Ruhnau, «How flexible electricity demand stabilizes wind and solar market values: The case of hydrogen electrolyzers,» Applied Energy, n° %1doi:10.1016/j.apenergy.2021.118194, 2021.
- Diana Böttger and Philipp Härtel, «On Wholesale Electricity Prices and Market Values in a Carbon-Neutral Energy System,» n° %1arXiv:2105.01127 [econ, q-fin], 2021.
- https://antares-simulator.org/
- J. Mays and J. D. Jenkins, «Financial Risk and Resource Adequacy in Markets with High Renewable Penetration,» IEEE Transactions on Energy Markets, Policy and Regulation , n° %1https://doi.org/10.1109/TEMPR.2023.3322531, 2023.
- European backbone
